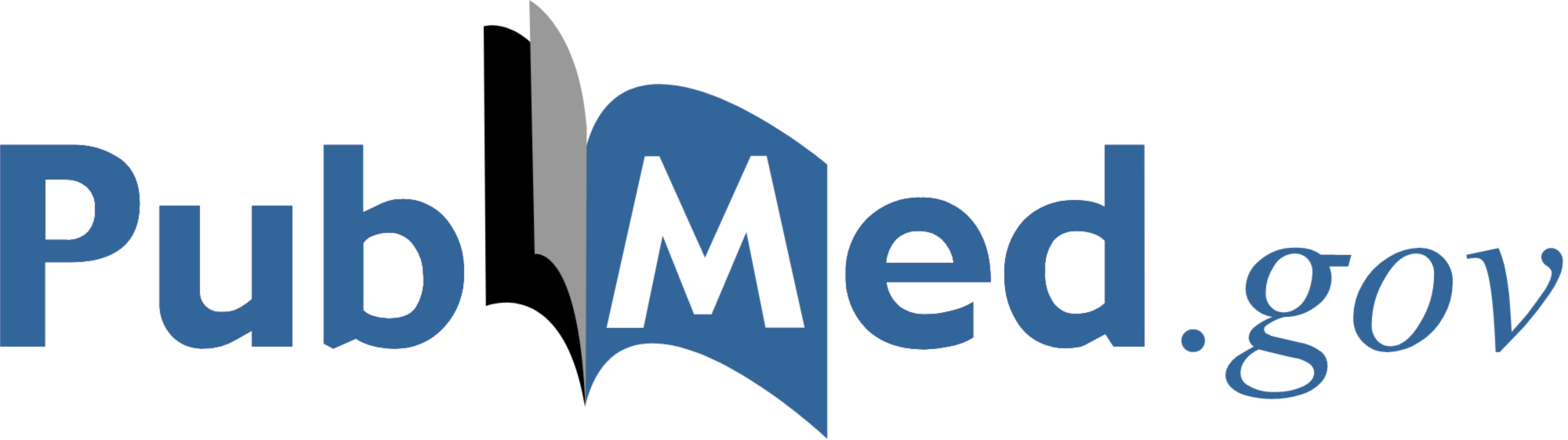
Journal List > Elsevier Public Health Emergency Collection > PMC9482090

Associated Data
Abstract
Cannabidiol (CBD) was formulated as a metered dose inhaler (CBD-MDI) and evaluated in vitro for its efficacy as an inhaled dosage form against inflammation caused by the SARS-CoV-2 virus, lipopolysaccharide (LPS) from Escherichia coli, silica particles, nicotine, and coal tar. A CBD-MDI formulation was prepared with 50 mg of CBD in 10 mL for a CBD dose of 250 μg/puff. The formulation ingredients included CBD, absolute ethanol as a cosolvent, and HFA-134a as the propellant. High aerosol performance of CBD-MDI was obtained with mass median aerodynamic diameter of 1.25 ± 0.01 μm, geometric standard deviation of 1.75 ± 0.00, emitted dose of 244.7 ± 2.1 μg, and fine particle dose of 122.0 ± 1.6 μg. The cytotoxicity and anti-inflammatory effectiveness of CBD-MDI were performed in alveolar macrophage (NR8383) and co-culture of alveolar macrophage (NR8383) and human lung adenocarcinoma (A549) cell line. CBD delivered from an MDI was safe on respiratory cells and did not trigger an immune response in alveolar macrophages. CBD-MDI effectively reduced the generation of cytokines in immune cells treated with viral antigen S-RBD, bacterial antigen LPS, silica particles, and coal tar. The efficacy of CBD-MDI was comparable to budesonide. Furthermore, the findings demonstrated that the use of CBD-MDI was more effective in treatment rather than prevention when inflammation was induced by either a viral or bacterial stimulant.
Abbreviations
- HFA-134a
- 1,1,1,2-tetrafluoroethane
- MTT
- 3-(4,5-dimethylthiazol-2-yl)-2,5-diphenyltetrazolium bromide
- EtOH
- absolute ethanol
- ACE 2
- angiotensin-converting enzyme 2
- CBD
- cannabidiol
- CB1
- cannabinoid 1 receptor
- CB2
- cannabinoid 2 receptor
- COVID-19
- coronavirus disease 2019
- ED
- emitted dose
- ELISA
- enzyme-linked immunoassay
- FPD
- fine particle dose
- FPF
- fine particle fraction
- HPLC
- high-performance liquid chromatography
- IL-1β
- interleukin 1β
- IL-6
- interleukin-6
- LPS
- lipopolysaccharide
- MMAD
- mass median aerodynamic diameter
- MDI
- metered dose inhaler
- NGI
- Next Generation Impactor
- pMDI
- pressurized metered dose inhaler
- S-RBD
- SARS-CoV-2 spike receptor binding protein
- SARS-CoV-2
- severe acute respiratory syndrome coronavirus 2
- TNF-α
- tumor necrosis factor-alpha
- USP
- United States Pharmacopeia
- AMs
- alveolar macrophages
1. Introduction
In humans, the respiratory tract is responsible for the life-sustaining process of gas exchange. However, the airways are extremely vulnerable to pathogens, such as severe acute respiratory syndrome coronavirus 2 (SARS-CoV-2), and environmental pollutants, such as particles ≤2.5 μm (PM 2.5) and cigarette smoke, which are the leading causes of respiratory disease [1]. The coronavirus infectious disease 2019 (COVID-19) pandemic has posed a serious health danger to the global population. COVID-19 caused by the SARS-CoV-2 virus is transmitted to healthy people through tiny droplets released from coughs, sneezes, speaking or exhaling, personal contact (shaking hands) and touching contaminated objects [2]. Infections arise when the droplets are breathed into the respiratory tract and into the lungs and can range from mild to severe acute respiratory syndrome. Upon the binding of SARS-CoV-2 spike glycoprotein to the angiotensin-converting enzyme 2 (ACE 2) receptors on alveolar epithelial cells, a cascade of molecular processes is initiated. This ultimately culminates in hyperinflammation. In COVID-19, the immune response has been linked to intensive care unit hospitalizations and death. These immunological responses in severe COVID-19 could be prognostic of a cytokine storm with undesirable clinicopathological outcomes [3]. In patients with a serious COVID-19 infection that leads to mortality, a strong correlation exists with elevated levels of serum complement components (C3a and C5a), inflammatory cytokines, and the chemokine interleukin-8 (IL-8). As a result, reducing excessive complement mediators as well as pro-inflammatory cytokines and chemokines may be useful therapeutic strategies against COVID-19 disease [4].
Aside from infection, pollutants and toxicants, such as PM 2.5 particles and cigarette smoke, can produce oxidative stress, which can lead to respiratory illness and severe inflammation. Excessive production of reactive oxygen species elicits high secretion of inflammatory cytokines, increased expression of inflammatory markers, and severe inflammatory damage. A number of inflammatory factors, such as interleukin-1β (IL-1β) and tumor necrosis factor-α (TNF-α), are synthesized and activated as a result of cytokine activation. Particles with an aerodynamic diameter ≤2.5 μm are related to an elevated risk of respiratory system and circulatory disease morbidity and death. PM 2.5 is very harmful to human health due to its small size and powerful penetrating capabilities [5]. In addition, one of the most common causes of lung inflammation is the large amounts of nicotine and coal tar in cigarette smoke [6]. Since inflammation aggravates the degenerative process of respiratory disorders, inhibiting the inflammatory response is an effective strategy to mitigate disease.
Cannabinoids are chemical components found in the Cannabis sativa plant. 9-tetrahydrocannabinol and cannabidiol (CBD) are the two most studied cannabinoids [7]. CBD is a non-psychotropic cannabinoid, which was reported to have beneficial properties against neuropsychiatric disorders, brain inflammatory diseases, colitis, sepsis-related encephalomyelitis, and inflammatory lung disease [8]. The human cannabinoid system is well known, and the most common receptors are CB1 and CB2. CB1 receptors are mostly located in the brain and central nervous system with some in other organs. CB2 receptors are found primarily in peripheral organs, in particular the cells of the immune system. CBD activates CB1 and CB2 receptors, which have anti-inflammatory, immunomodulatory, and analgesic properties. The CB2 receptor controls the release of inflammatory cytokines, which modulates the immune system [9,10]. One putative means of immunological control by cannabinoid during inflammation is by inhibiting cytokine generation by the immune cells and their modulation of the well-regulated immune response [8]. Consequently, it is expected that delivery of CBD to the lungs can be beneficial in alleviating inflammation. Furthermore, a new study revealed early evidence that CBD may help to reduce cytokine storms and excessive lung inflammation in patients with COVID-19 [11]. Other studies indicated that CBD improved outcomes in COVID-19 patients [[12], [13], [14]]. Clinical reports have indicated that a cytokine storm is associated with acute respiratory distress syndrome and is the leading cause of mortality in severe cases of COVID-19. CBD decreases acute respiratory distress syndrome by reducing the levels of inflammatory cytokines, thereby limiting damage in the lung and improving the functional capacity of airways leading to increased oxygen levels [15].
Inhaling CBD directly into the lungs is the most effective way to treat lung inflammation because the respiratory system is the primary route of infection, pollution, and toxicant exposure, as well as the site of disease progression. The International Society for Aerosols in Medicine has also called for the development of inhaled therapies for COVID-19 treatment because the symptoms of COVID-19 are mainly manifested in the respiratory system [16,17]. Currently, the inhalation delivery of drugs to the lungs is the most important route of administration for lung diseases. Furthermore, local delivery of drugs to the lungs may allow maximum pharmacological targeting with minimum systemic exposure [16].
The objective of this research was to produce CBD in an aerosol dosage form for delivery directly to the lungs to reduce inflammation caused by the SARS-CoV-2 spike receptor binding domain (S-RBD), lipopolysaccharide (LPS) from Escherichia coli, silica particles, nicotine, and coal tar. Pressurized metered dose inhaler (pMDI) was employed using non-polar propellant as the dispersion medium. Given that CBD is highly oil-soluble, it would therefore be most suitable as an pMDI formulation. The schematic diagram of this study is shown in Fig. 1 .
Schematic diagram of the feasibility of cannabidiol metered dose inhaler to target the cannabinoid receptor type 2 that is a possible target in SARS-Co-V2 infection.
2. Materials and methods
2.1. Materials
A CBD sample was provided gratis by AVS Innovation (Bangkok, Thailand) with 99% purity. A CBD standard was purchased from the Department of Medical Sciences, Ministry of Public Health (Bangkok, Thailand). Analytical grade absolute ethanol (EtOH) and acetonitrile were acquired from RCI Labscan (Bangkok, Thailand). Pharmaceutical grade (99.9%) of 1,1,1,2-tetrafluoroethane (HFA-134a) was purchased from Mexichem Fluor (Runcorn, UK). Recombinant S-RBD was obtained from the National Biotechnology Center, NSTDA, Ministry of Higher Education Science Research and Innovation, Thailand. Silica, which is normally used as a packing material in high-performance liquid chromatography (HPLC) systems, was available as 2.5 μm spherical particles (Hypersil, ThermoFisher Scientific, MA, USA). Nicotine was obtained from Sigma Aldrich, MO, USA. Coal tar was from P.C. Drug Center Co. Ltd., Bangkok, Thailand.
2.2. Preparation of CBD metered-dose inhalers (CBD-MDIs)
The MDIs consisted of three main ingredients, namely CBD, EtOH, and HFA-134a. CBD was the active pharmaceutical ingredient. EtOH served as a co-solvent to dissolve CBD or disperse CBD before adding the propellant in the final step in order to transfer the active ingredient in the canister. HFA-134a served as a non-aqueous propellant. CBD in this MDI dosage form was 250 μg per puff and the MDI contained 200 doses in 10 mL; therefore, the CBD content in each MDI container was 50 mg. The solubility of CBD in ethanol is approximately 35 mg/mL [18]. Thus, it was expected that CBD would be completely dissolved in about 1.5 mL of ethanol or less than 1.5 mL when using the propellant as a co-solvent since CBD is readily soluble in HFA-134a [19]. The compositions for the preparation of the CBD-MDIs are presented in Table 1 . To prepare the formulation, CBD was accurately weighed and the required amount of EtOH was added to produce the product concentrate. The CBD product concentrate was dispensed into MDI glass canisters that were not coated on the inside (Schott AG, Mainz, Germany). The canisters were then promptly sealed with metering valves (Bespak Europe, Ltd., Norfolk, UK) and an aerosol crimping machine (model 2016, Pamasol Willi Mäder, Zurich, Switzerland). Using an aerosol filling machine (Pamasol Willi Mäder AG, Zurich, SZ), HFA-134a (9 mL for formulation F1 and 7.5 mL for formulation F2) was loaded into the canisters after capping. After that, the MDIs were well mixed and kept at room temperature. The finished MDI canister was fitted with an actuator (Aerocare Co. Ltd., Bangkok, Thailand) to actuate the valve to release the content from the MDI.
Table 1
Formulations of cannabidiol metered dose inhaler (CBD-MDI).
Ingredients | Formulation and amount
|
Function | |
---|---|---|---|
F1 | F2 | ||
Cannabidiol (CBD) | 50.0 mg | 50.0 mg | Active ingredient |
Absolute Ethanol | 1.0 mL | 2.5 mL | Co-solvent |
HFA-134a qs. to | 10.0 mL | 10.0 mL | Propellant |
2.3. Chemical analysis of CBD
CBD was analyzed by an HPLC system (CBM-20A; Shimadzu Corporation, Tokyo, Japan) that consisted of an HPLC pump (LC-30AD), autosampler (SIL-30AC), PDA detector (SPD-M20A), and column oven (CTO-20AC). The separations were performed on a C18 reverse-phase column (150 mm × 2.1 mm, 3 μm, Hypersil BDS; Thermo Scientific, MA, USA) at 15 °C. The mobile phase consisted of a degassed mixture of 30% acetonitrile and 70% ultrapure water. The mobile phase was used to dissolve the standard or sample solution. The injection volume was 20 μL. The flow rate was set at 0.5 mL/min. The separation was monitored spectrophotometrically at 207 nm. The acquired data was processed using LC solution software (Shimadzu Corporation, Tokyo, Japan). The validation method included specificity, limit of detection, limit of quantitation, accuracy, precision, linearity, and robustness. The HPLC analytical system was very specific to CBD with 3.7 min retention time, the limit of CBD detection was 0.25 μg/mL, limit of quantitation was 1 μg/mL. The accuracy and precision of analytical system were 99% and 1.2% relative standard deviation. The HPLC system gave linearity over 10–50 μg/mL, and the system is robust enough for different operators and HPLC systems.
2.4. In vitro evaluation of aerosol characteristics
The mass median aerodynamic diameter (MMAD), emitted dose (ED), fine particle dose (FPD) and fine particle fraction (FPF) were evaluated using the Next Generation Impactor (NGI; MSI Corporation, MN, USA) without a pre-separator. The NGI was connected to a vacuum pump with a flow rate of 30 L/min. The evaluated dose was 1 puff of the MDI sample (equivalent to 250 μg of CBD). The MDI was shaken for 10 s before each actuation and the first dose was discharged to waste. In order to collect the sample, the metal inlet and all stages of NGI were rinsed with 10 mL of the mobile phase in each stage. The ED and FPD were then determined by the HPLC method described above and the MMAD and FPF were calculated. The FPD refers to the dose of the aerosolized drug particles with an aerodynamic diameter smaller than 4.0 μm (stage 3), and FPF is the ratio of the FPD to ED. The MMAD is defined as the diameter at which 50% of the particles by mass are larger than the median aerodynamic diameter, and another 50% are smaller than the median aerodynamic diameter. The experiment was repeated at least three times.
The assay and delivered-dose uniformity of the CBD-MDI were performed according to United States Pharmacopeia (USP) general chapter <601> [20]. Briefly, for content uniformity determination, three doses of CBD content were collected at the beginning of the total dose (3rd dose to 5th dose), four doses in the middle (100th dose-103rd dose), and three doses at the end (198th dose to 200th dose) using a dose collecting apparatus as described in the USP. The amount of CBD was determined for each of the three separate containers by HPLC according to the method described in Section 2.3.
2.5. Recombinant spike receptor binding domain (S-RBD) production
The coding sequence for the S-RBD of SARS-CoV-2 (S-RBD, residue 317–539) was optimized for expression in mammalian cells from the sequence obtained from the reference strain (Wuhan-Hu-1) and cloned into pSecTag using restriction enzyme cloning (BsiWI and XhoI) in-frame with the Igκ leader sequence at the N-terminus and the Myc-His tag at the C-terminus. Recombinant tagged S-RBD protein was produced according to a previously published protocol [21]. Briefly, human embryonic kidney 293T cells were maintained in OptiMEM with 10% fetal bovine serum supplement and transfected with pSecTag-SRBD using FuGENE transfection reagent according to the manufacturing’s protocol. The cell media were then changed to OptiMEM with no supplement at 6 h post-transfection. At 72 h post-transfection, the supplement was conditioned with 4X binding buffer (1.2 mM NaCl, 200 mM NaH2PO4, 40 mM imidazole). Then, the conditioned supernatant was mixed with Ni-NTA agarose resin (Qiagen) for 1 h. The unbound proteins were washed off with the wash buffer (300 mM NaCl, 50 mM NaH2PO4, 20 mM imidazole). Recombinant S-RBD was then eluted with the elution buffer (300 mM NaCl, 50 mM NaH2PO4, 250 mM imidazole). The eluted fractions were pooled, concentrated and buffer-exchanged into PBS using a 10 molecular weight cut-off centrifugal filter unit. The protein concentration was measured using the Bradford method [22]. Recombinant S-RBD was stored at −80 °C in small aliquots prior to use.
2.6. Cell culture conditions
2.6.1. Alveolar macrophage cell line (NR 8383)
The rat alveolar macrophage cell line (NR 8383, ATCC CRL-2192, MD, USA) was cultured in complete media containing F-12 Kaighn’s medium (Gibco®, USA) supplemented with 15% fetal bovine serum (FBS, Gibco®, USA), and 100 U/mL penicillin/streptomycin (Gibco®, USA). The cells were incubated at 37 °C in a 5% CO2 incubator and the medium was changed every two days. The cells were harvested by gentle shaking, followed by the addition of fresh complete media to create a new single-cell suspension for further incubation.
2.6.2. Human lung adenocarcinoma cell line (A549)
The human lung adenocarcinoma cell line (A549, ATCC: CCL185, MD, USA) was cultured in complete media containing Kaighn’s Modification of Ham’s F-12 Medium (F–12K, Gibco®, USA) containing 10% fetal bovine serum (FBS, Gibco®, USA) and antibiotics (100 U penicillin and 100 U/mL streptomycin, Gibco®, USA) under 5% CO2 at 37 °C. The media were changed every alternate day. When the cells reached confluence, they were harvested using 0.25% trypsin-EDTA (Gibco®, USA), followed by the addition of fresh complete media to create a new single-cell suspension for further incubation.
2.6.3. Co-culture of alveolar macrophage (NR8383) and human lung adenocarcinoma cell line (A549)
The NR8383 and A549 cell lines were co-cultured at a ratio of 1:1 in the same complete media (F-12 Kaighn’s medium supplemented with 15% FBS and 100 U/mL penicillin/streptomycin) in a 5% CO2 incubator at 37 °C. The media were changed every alternate day. When the cells reached confluence, they were harvested using 0.25% trypsin-EDTA (Gibco®, USA), followed by the addition of fresh complete media to create a new single-cell suspension for further incubation.
2.7. Cell proliferation and viability assay
The NR8383 alone or the co-cultured NR8383-A549 cell lines were seeded in a 96-well plate using 50 μL of 1 × 105 cell/mL in complete media per well. The plate was immediately incubated at 37 °C under 5% CO2 for 24 h. Fresh complete cell culture medium was used to dissolve the CBD, CBD-MDI formulations, nicotine, coal tar and disperse 2.5 μm silica particles. In order to prepare the CBD-MDI sample, 1 puff of CBD-MDI (F1) was sprayed into each well of a 6-well plate with 3 cm of distance and allowed to completely evaporate. The exact CBD concentration in each well was determined using the HPLC technique outlined in section 2.3. The CBD samples were serially diluted in complete cell culture media to produce the appropriate sample concentrations, and 100 μL of sample at various concentrations were introduced into each well. Untreated cells served as a negative control. MDI formulation without CBD was used as a blank sample. The viability of the cells following exposure to the samples at various concentrations was assessed using the 3-(4,5-dimethylthiazol-2-yl)-2,5-diphenyltetrazolium bromide (MTT) assay after a 24 h incubation period. Briefly, the cell supernatants were removed and replaced with 80 μL of fresh media and 20 μL of MTT solution (5 mg/mL). The well plates were further incubated at 37 °C under 5% CO2 for 4 h. The media was removed from the well plates and 100 μL of dimethylsulfoxide was added to dissolve the forming formazan salt from the viable cells. The absorbance of dissolved formazan salt was determined by a microplate reader (Biohit 830, Biohit®, Helsinki, Finland) at a wavelength of 570 nm. The percentage of cell viability was calculated and compared to a negative control.
2.8. Determination of cytokine response
2.8.1. Cell culture conditions and sample treatment
The cells were seeded into 96-well plates in a volume of 100 μL of 105 cells/mL and allowed to grow until they were 80% confluent. S-RBD (10 μg/mL) or Escherichia coli LPS (1 μg/mL) was employed to trigger in vitro lung cell inflammation in both NR8383 cells and co-cultured NR8383-A549 cells, whereas 2.5 μm silica particles, nicotine, or coal tar, at concentrations of 60, 1, and 40 μg/mL, respectively, was used in NR8383 cells only.
The ability of CBD-MDI (F1) to reduce cytokine production in inflamed alveolar macrophages stimulated by various inflammatory stimuli was examined. CBD-MDI delivered to the well was diluted by one-fifth to achieve the desired concentration used to examine. Budesonide (50 μg/mL) was used as a positive anti-inflammatory agent. As indicated in Fig. 2 , cell exposures to each inflammation stimulant and sample (CBD-MDI or budesonide) occurred in four distinct orders: pre-treatment, loading-washout, treatment, and co-administration.
All modes of experimentation (Pre-treatment, Treatment, Loading-Washed out, and co-administration) were designed as follows: Pre-treatment mode: Cells were treated with CBD-MDI for 24 h before exposure to inflammatory stimuli. Treatment mode: Cells were initially challenged to inflammatory stimuli 24 h before being given CBD-MDI. Loading-Washed out mode: Cells were exposed to CBD-MDI for 24 h before being flushed out and then challenged with inflammatory stimuli. Co-administration mode: inflammatory stimuli and CBD-MDI were given to cells at the same time for 24 h.
The levels of generated cytokines were determined using the enzyme-linked immunoassay (ELISA) technique described below, and the results of all experiments were compared.
2.8.2. Cytokine assay
TNF-α, IL-1β, and interleukin-6 (IL-6) in cell supernatant were determined using the rat ELISA assay kit (R&D Systems, MN, USA). TNF-α, IL-1β or IL-6 diluents (50 μL) were added to each well. Then, 50 μL of experimental cell supernatant was added to the wells and incubated for 2 h at room temperature. Each well was washed five times with a buffer solution followed by addition of either 100 μL of TNF-α, IL-1β or IL-6 conjugate to each well. The plate was incubated for another 2 h. Then, each well was washed five times with buffer (400 μL), and 100 μL of substrate solution was added. The plates were incubated for 30 min at room temperature and 100 μL of stop solution was added. The reaction was recorded quantitatively at 450 nm based on a standard curve of TNF-α, IL-1β or IL-6.
3. Results and discussion
3.1. Formulation development and content of CBD delivered from the MDI
CBD, like other cannabinoids, is a non-polar compound that is insoluble in water (0.1 μg/mL) [23]. From the literature, the solubility of CBD in ethanol is 35 mg/mL [18] and freely soluble in non-polar liquids such as the HFA-134a propellant [19]. The CBD as an inhaler formulation was prepared using ethanol (1 mL or 2.5 mL) as a solvent to produce product concentrate and is miscible with HFA-134a propellant. CBD (50 mg) partially dissolved in 1 mL ethanol, but it was completely dissolved after the propellant was added. Consequently, both formulations were dissolved completely and clear solutions were obtained. Upon actuating the inhaler, the propellant rapidly evaporated, leaving only CBD to penetrate and deposit in the respiratory system. The small amount of ethanol, which is a low vapor pressure material, does not affect the performance of drug delivery to the airways.
The rationale of the specified dose of CBD in MDI was 250 μg/puff calculated from the pharmacokinetic data of CBD. Maximum plasma concentration (Cmax) and area under the curve of oral administration appeared to be dose dependent [24], and pharmacokinetic profiles of inhaled drug administration were similar to intravenous administration [25,26]. Several systematic reviews indicated that daily doses of CBD were highly variable, for example, between <1 and 50 mg/kg/d and 150–600 mg/d. Thus, it was quite difficult to justify a suitable daily dose [24,[27], [28], [29]]. In a recent phase 1 clinical study of CBD dry powder inhaler, the authors reported a Cmax of 18.78 ng/mL of CBD [30], and in general the lung drug concentration should be about 100-fold higher than the concentration in the plasma [31]. Also, it is worth noting that the extravascular lung fluid according to various reports is about 2–10 mL [32,33]. Thus, in our estimation we used 10 mL of lung fluid for the calculation. The plasma drug concentration of 18.78 ng/mL was multiplied by 100 to obtain a drug concentration deposition in the lung and multiplied by 10 mL of lung fluid. CBD deposition in the lower lung was estimated to be 18.78 μg. The bioavailability of CBD after smoking cannabis is reported to be 31% [24]. Therefore, the initial dose of inhalation of CBD is 18.78 μg divided by 31% = 60.6 μg. Typically only 20–25% of the emitted dose of a pMDI reaches the target site [34] but we chose 25% for this calculation; therefore, the calculated dose was 242 μg/dose. To make it easier to calculate, we specified a dose at 250 μg/dose.
From the CBD-MDI, the two formulations were assayed to obtain the percent labeled amount. Quantities of 101.6% and 100.1% were obtained in formulations F1 and F2, respectively. The CBD uniform delivered dose of both formulations was higher than 91%, which is acceptable according to the USP recommends 80–120% of the expected amount of 250 μg per actuation [20].
3.2. Aerosol properties of the CBD-MDI
The results of aerosol characterization of the CBD-MDI formulation using the NGI at a flow rate of 30 LPM is presented in Table 2 . The CBD deposition in each stage of NGI is shown in Fig. 3 . The deposition of CBD in F1 and F2 was around 50% in the USP inlet which is the highest amount in comparison with other stages which may indicate that large amount of CBD is likely to lost in the oral cavity. Whereas, stages 1–3, there are very low amount of CBD detected (less than 5%) from both formulations. From stages 3–7, the size distribution of CBD was obtained with the high amount of CBD on stages 5 and 6 which may predict that the CBD is likely to reach the small airways. The CBD-MDI has acceptable characteristics of an inhalation dosage form. The emitted doses of both formulations were high, especially formulation F1, which was close to the theoretical dose of 250 μg. This may have resulted from the high vapor pressure of the propellant that provided a high energy release of the drug from the container due to the lower content of ethanol. Ethanol concentration can influence the delivery characteristics of MDIs in three ways: (1) by changing the formulation density and thus changing the total mass of formulation atomized during actuation of the device, (2) by changing atomization of the formulation and the size of the atomized droplets, and (3) by changing the evaporation rate of these droplets towards their residual particle sizes [35]. The mass median aerodynamic diameter was 2.37 μm with a fine particle fraction of 43%–45%. Therefore, the drug is likely to reach the lower airways. All parameters except the assay contents of formulations F1 and F2 showed a significant difference (P < 0.05). Formulation F1 seemed to be more effective than formulation F2.
Table 2
The aerosol properties of CBD-MDIs (mean ± SD, n = 3).
Test | F1 | F2 | P-value |
---|---|---|---|
Assay (%Labeled claim) | 101.6 ± 1.58 | 100.1 ± 0.64 | >0.05 |
Content uniformity | 95.4 ± 2.09 | 91.4 ± 1.39 | <0.05 |
MMAD (μm) | 1.25 ± 0.01 | 1.54 ± 0.01 | <0.05 |
GSD | 1.75 ± 0.00 | 2.05 ± 0.10 | <0.05 |
ED (μg) | 244.7 ± 2.1 | 223.3 ± 1.3 | <0.05 |
FPF (%) | 49.8 ± 0.4 | 44.6 ± 1.2 | <0.05 |
FPD (μg) | 122.0 ± 1.6 | 99.6 ± 2.4 | <0.05 |
Abbreviation: Mass median aerodynamic diameter (MMAD), emitted dose (ED), fine particle fraction (FPF), fine particle dose (FPD) and geometric standard deviation (GSD).
Cannabidiol deposition (%, mean ± SD, n = 6) in each stage of next-generation cascade impactor (NGI) experiments at an airflow rate of 30 L/min. The NGI stage cutoff diameters are as follows: stage 1 (11.72 μm), stage 2 (6.40 μm), stage 3 (3.99 μm) stage 4 (2.30 μm) stage 5 (1.36 μm) stage 6 (0.83 μm) stage 7 (0.54 μm).
From the results in Table 2, formulation F2 seemed to have higher content uniformity than formulation F1 (95.4 ± 2.09% vs. 91.4 ± 1.39%) due to lower variation. Additionally, the FPF for formulation F1 was 49.8 ± 0.4 whereas that for formulation F2 was 44.6 ± 1.2%. The fine particle mass decreases when ethanol is added for the same drug concentration as similar to several previous study [35,36]. Thus, when the concentration of ethanol is increased, the overall delivery efficiency of the formulation decreases, thereby limiting effectiveness of using ethanol as a solubilizing aid [35]. The low content of ethanol in formulation F1 may have an effect on vapor pressure in the MDI system. When discharged from the pressurized container, the propellant will evaporate and release the drug from the valve. If the formulation contains a high content of ethanol, the atomized droplets is larger and evaporate more slowly than droplets containing less ethanol or only propellant [35,37]. As the concentration of ethanol increases, the vapor pressure of the formulation decreases, which in turn affects the atomization process [35]. The ethanol concentrations in this investigation are 10% (F1) and 25% (F2). However, the highest percentage of ethanol approved in commercial pMDI is 95.89% [35]. For the development of CBD, pMDI in this study was chosen to use as low as possible because it affects aerosol performance. The previous clinical study of THC-CBD inhalation solution pMDI in HFA227 used high content of ethanol in the formulation and showed pharmacokinetic properties similar to injection preparation [26]. So that the content of ethanol may vary when the aerosol performance and clinical outcomes reach the expected target.
3.3. Response of respiratory cells to CBD delivered from an MDI
The safety profiles of CBD and CBD-MDI (F1) in contact with respiratory cells, including the NR8383 and co-cultures of NR 8383-A549, were determined. The NR8383 and A549 cells were co-cultured in the same culture media and environment. The A549 adhered to the well plate, whereas some NR8383 adhered together with the A549 and some floated in the media. In reference with the sample preparation technique for the CBD-MDI cytotoxicity test, the inhaler device was actuated to deliver CBD directly to a well of a 6-well plate. The exact amount of drug delivered to the well was then assessed to obtain the actual concentration of the tested drug. The results indicated that only 176.23 ± 5.25 μg or about 71.69% of total amount of CBD reaching the well plate.
The percentages of cell viabilities after exposure to CBD and CBD-MDI (F1) at various concentration are depicted in Fig. 4 . Pure CBD toxicity was found at concentrations greater than 31.25 μg/mL. However, nearly 100% cell survival was observed at CBD concentrations <16.63 μg/mL. The obtained viability results of both single and co-cultured cells followed similar pattern. In contrast, CBD’s cytocompatibility improved when formulated as an MDI. More than 95% cell viability was achieved for both NR8383 and co-cultured NR8383-A549 with the MDI formulation containing CBD equivalent to 44.81 μg/mL. However, when the cells were subjected to the same concentration of pure CBD, the viability of both NR8383 and co-cultured of NR8383-A549 decreased to less than 20%. It is uncertain how CBD manufactured as an MDI is less toxic to the cells. This would be as a result of the formulation’s ingredients, which included ethanol and the propellant HFA-134a, causing CBD to dissolve, therefore improved CBD’s cytotoxicity profile.
Cell viability percentages of NR 8383 (black bar) and co-cultured NR8383-A549 (white bar) after treatment with raw CBD and CBD-MDI at different concentrations. Data expressed as a mean ± SD, n = 4.
3.4. CBD-MDI ability to mitigate the effects of cytokine generated by S-RBD or LPS stimulated NR8383 and co-cultured NR8383-A549
The viral protein SARS-CoV-2 S-RBD is responsible for attaching to the host receptor ACE 2 in order to enter the target cell [38,39]. LPS is widely known as an endotoxin and is the most common component of the outer membrane of gram-negative bacteria. In this investigation, the S-RBD and E. coli LPS were used to stimulate the NR8383 and co-cultured NR8383-A549 cell lines to produce the pro-inflammatory cytokines TNF-α, IL-1β, and IL-6 (Fig. 5 and Fig. 6 ).
TNF-α, IL-1β and IL-6 levels generated by NR8383 and co-cultured NR8383-A549 after inflammation was induced with S-RBD. CBD-MDI or Budesonide treated to the cell in three distinct separation studies (Pre-treatment, Loading-washout and Treatment) with presented in each plot. Data expressed as a mean ± SD, n = 4. The symbol * denotes that the compared data were significantly different (p-value < 0.05).
TNF-α, IL-1β and IL-6 levels generated by NR8383 and co-cultured NR8383-A549 after inflammation was induced with LPS. CBD-MDI was treated to the cell in three distinct separation studies (Pre-treatment, Loading-washout and Treatment) with presented in each plot. Data expressed as a mean ± SD, n = 4. The symbol * denotes that the compared data were significantly different (p-value < 0.05).
According to the safety profile of CBD-MDI formulation F1, a CBD concentration of less than 44 μg/mL was not toxic to respiratory cells; therefore, a one-fifth dilution of the CBD delivered dose, or approximately 35 μg CBD/mL, was used to evaluate its ability to inhibit S-RBD or LPS-induced immune responses. Treating the untreated or control cells with CBD-MDI resulted in generating cytokines in extremely low quantities (<100 pg/mL) (Figs. 5 and and6).6). This indicated that CBD-MDI alone did not induce the production of cytokines in immune cells. The efficiency of a CBD-MDI formulation in lowering cytokine production when AM cells were triggered with S-RBD or LPS was investigated in three different experimental modes that included pre-treatment, loading-washout, and treatment, as mentioned in section 2.8.1 and Fig. 2. When the AM cells were stimulated with S-RBD (Fig. 5) or LPS (Fig. 6), CBD-MDI was found to reduce all measured inflammation markers (TNF-α, IL-1β, and IL-6) in all experiment modes. The loading-washout study, on the other hand, revealed that CBD binding to the receptor was reversible since washing CBD-MDI from the cell surface resulted in lower anti-inflammatory activity, which was inadequate to suppress the cytokine level produced by S-RBD or LPS stimulated AMs. The large amount of cytokine produced in loading-washout mode was equivalent to that produced by an S-RBD or LPS-stimulated macrophage as a control (Figs. 5 and and6).6). More study on the receptor binding affinity of CBD, however, is essential.
The potential of the CBD-MDI formulation to protect the cells from the action of S-RBD was compared to budesonide as the positive control (Fig. 5). Since budesonide is a glucocorticosteroid that is an efficient immunomodulator, it decreases cytokine patterns and alleviates allergic rhinitis symptoms [40]. In this investigation, 50 μg/mL budesonide was used as a positive control, along with the same three experimental treatments. The CBD-MDI formulations gave results equivalent to budesonide with significantly lower levels of some of the studied cytokines (Fig. 5). Furthermore, the findings revealed that CBD-MDI and budesonide were more effective when given after 24 h of S-RBD activation (treatment mode, Fig. 5).
CBD in the CBD-MDI formulations was shown to inhibit cytokine production in immune cells induced by LPS (Fig. 6). CBD-MDI used as a pre-treatment strategy or before stimulus exposure gave less cell protection. However, when CBD-MDI was employed in a treatment mode after the inflammatory stimulant exposure, there was a significant reduction in the levels of bacterial antigen stimulated inflammatory mediators.
CBD has a very low affinity to the cannabinoid receptor, indicating a weak interaction with the receptor [41]. Furthermore, CBD’s anti-inflammatory effect is reversible, as evidenced by the loading washout experimental mode, once CBD is removed. In order to protect the cells from inflammatory stimulant or pre-treatment mode, cells were exposed to CBD-MDI for 24 h prior to being primed with S-RBD or LPS to stimulate inflammatory reactions. However, due to the low affinity between CBD and CB2 receptors, the interaction of them is insufficiently to elicit anti-inflammatory effects against S-RBD or LPS stimulation delivered 24 h later. In contrast, in the treatment mode, S-RBD or LPS was given to induce inflammation in the cell prior to CBD-MDI administration. Despite the weak interaction, the immediate anti-inflammatory effect that followed CBD-MDI binding to the CB2 receptor was able to lower cytokine production from inflamed cells more effectively than the pre-treatment strategy.
3.5. Safety profiles of pollutants (PM 2.5) and toxicants (nicotine, coal tar) to the respiratory cells
The lungs are directly exposed to environmental antigens that include pathogens, pollutants, and toxins such as particulate matter of ≤2.5 μm and tobacco smoke. Nicotine and coal tar are hazardous components from cigarette smoke. PM 2.5 can produce oxidative stress, which can lead to respiratory illness and severe inflammation. Cigarette smoke causes inflammation by increasing the concentration of immune cells in the airway and triggering the production of pro-inflammatory cytokines such as TNF-α, IL-1β, IL-6, chemokine IL-8, and granulocyte-macrophage colony-stimulating factor [42].
An MTT assay was used to evaluate the viability of NR 8383 cells after exposure to 2.5 μm silica particles, nicotine, and coal tar (Fig. 7 ). The results revealed that the cells could survive after short-term exposure to 2.5 μm silica particles when the concentration was lower than 125 μg/mL. However, long-term exposure may give different results. Nicotine exhibited considerable toxicity to NR 8383 cells at concentrations higher than 1.6 μg/mL. Cell death was not observed at any of the tested concentrations of coal tar (2.5–40 μg/mL). Based on viability results, the concentrations of 2.5 μm silica particles, nicotine, and coal tar utilized to assess allergenic potential were 60, 1, and 40 μg/mL, respectively.
Percentage of NR8383 cell line viability when treated with silica particle of 2.5 μm, nicotine and coal tar at various concentrations. Data expressed as a mean ± SD, n = 4.
3.6. Allergenic potential of nicotine, coal tar, and 2.5 μm silica particles
The abilities of 2.5 μm silica particles, nicotine, and coal tar to elicit an immunological response in alveolar macrophages were assessed via the generated inflammatory markers that included IL-1β, IL-6, and TNF-α (Fig. 8 ). Nicotine at the tested concentration (1 μg/mL) mildly provoked AMs to produce a significantly higher level of IL-1β, IL-6, and TNF-α compared to the control (P < 0.05). When exposed to coal tar, the AMs produced much more IL-6 and TNF-α than the untreated control. Silica particles of 2.5 μm produced the strongest immunological response with cytokine levels of 300.00, 130.75, and 206.17 pg/mL for IL-1β, TNF-α, and IL-6, respectively. This meant that 2.5 μm silica particles were the most powerful allergen.
TNF-α, IL-1β and IL-6 levels generated by NR8383 after inflammation was induced with coal tar, nicotine or silica particle of 2.5 μm. CBD-MDI was treated to the cell in three distinct separation studies (Pre-treatment, Treatment and Co-administration) with presented in each plot. Data expressed as a mean ± SD, n = 4. The symbol * denotes that the compared data were significantly different (p-value < 0.05).
The ability of CBD-MDI to alleviate inflammation caused by nicotine, 2.5 μm silica particles, or coal tar was further investigated (Fig. 8). Pre-treatment, treatment, and co-administration were the three modes of studies used. Fig. 2 shows the order of the chemicals used to challenge the cells. The maximum CBD activity was achieved when the allergens and CBD-MDI were given simultaneously in co-administration mode.
In healthy airspaces, alveolar macrophages are the most common immune cell type [42]. Enhanced cytokine levels were induced and in vitro lung inflammation began shortly after the AMs were exposed to 2.5 μm silica particles, nicotine, and coal tar. Co-administration of allergens (2.5 μm silica particles, nicotine, and coal tar) and CBD-MDI may cause two challenging chemicals to compete for binding to the AM cell surfaces. However, when the cell surface was bound to CBD-MDI, an immune response was not triggered, which manifested in a lower level of cytokine production compared to when the AMs were challenged with one allergen only. Furthermore, CBD also has anti-inflammatory properties because it interacts with the CB2 receptor on immune cells [43]. Thus, CBD enhanced activities in reducing cytokine levels could be derived from these dual effects. Furthermore, similar to the conclusions drawn from the previous section, CBD-MDI was found to be more effective as a treatment rather than as a pre-treatment measure. This could be due to the low affinity of CBD for the CB2 receptor [43], which means its anti-inflammatory impact is not as potent or as long-lasting once the cells become exposed to the allergen. In the treatment mode, anti-inflammation was induced shortly after CBD was bound to the CB2 receptor, which retained the highest effects and demonstrated greater potency in reducing the effect.
Finally, the CBD-MDI formulations were found to potentially alleviate the effects of 2.5 μm silica particles, nicotine, and coal tar on AM cell line inflammation. CBD binding to the CB2 receptor was most effective when CBD was applied simultaneously with the allergens and more effective in the treatment mode compared to the pre-treatment mode.
4. Conclusion
CBD-MDI was effectively developed in this study using formulations that included 50 mg of CBD, absolute ethanol as a cosolvent, and volume adjustment to make 10 mL with HFA-134a propellant. The dose of CBD was 250 μg/puff. Both CBD-MDI formulations demonstrated high aerosol performance as recommended by the USP. CBD-MDI was found to be safe for respiratory cells and did not trigger an immune response when exposed to AMs. CBD MDI was not an irritant and is unlikely to provoke the production of inflammatory cytokines in the airways. CBD showed effectiveness in reducing the generation of cytokines in immune cells treated with a bacterial antigen (LPS), viral antigen (S-RBD), or air pollutants (2.5 μm silica particles and coal tar). The advantage of CBD-MDI is direct targeting to the CB2 receptor with a very low dose. CBD-MDI is expected to have a rapid onset in clinical settings. As such, under the current situation of SARS-CoV-2, CBD-MDI could be deployed to alleviate inflammation due to a cytokine storm. However, this work was carried only in an in vitro lung inflammation model that used related cell lines. Further studies are necessary before CBD MDI can be used clinically.
CRediT authorship contribution statement
Teerapol Srichana: Conceptualization, Methodology, Writing – original draft, Writing – review & editing, Project administration, Funding acquisition. Charisopon Chunhachaichana: Methodology, Investigation. Roongnapa Suedee: Conceptualization, Writing – original draft, Writing – review & editing. Somchai Sawatdee: Writing – original draft, Writing – review & editing, Visualization. Narumon Changsan: Writing – original draft, Writing – review & editing, Visualization.
Declaration of competing interest
The authors declare the following financial interests/personal relationships which may be considered as potential competing interests: Teerapol Srichana reports was provided by Prince of Songkla University. Teerapol Srichana has patent CANNABIDIOL METERED DOSE INHALERS FOR PROTECTION OF COVID ANTIGEN AND OTHER ALLERGENS pending to Teerapol Srichana.
Acknowledgments
This research was supported by the National Science, Research and Innovation Fund and Prince of Songkla University (PHA 6405087S). The authors would like to thank the Drug Delivery System Excellence Center, Faculty of Pharmaceutical Sciences, Prince of Songkla University for providing the facilities and the National Biotechnology Center (BIOTEC), NSTDA, Ministry of Higher Education Science Research and Innovation, Thailand for providing the spike protein of SARS-CoV-2, as well as Ms. Titpawan Nakpheng for technical support.