Plant flavonoids in cancer chemoprevention: role in genome stability
1. Introduction

______
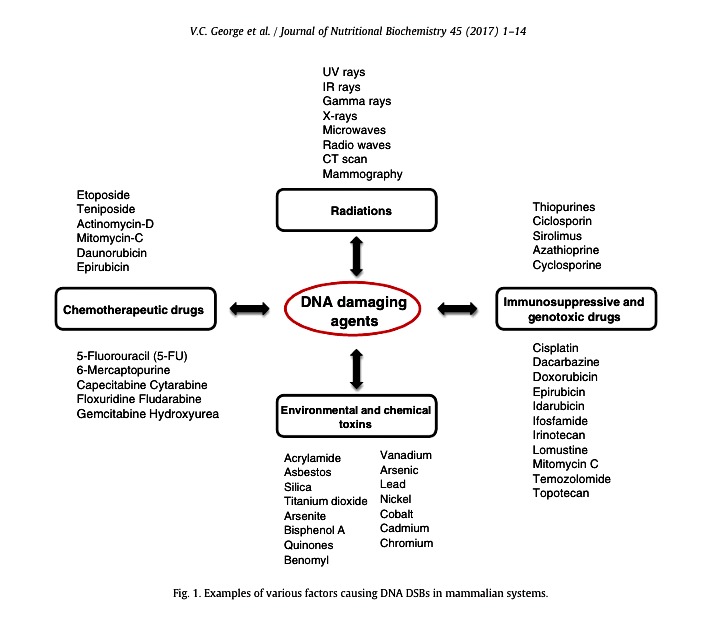

Fig. 2. Cellular mechanisms of flavonoids on DNA damage and repair. Different DNA-damaging agents may form DSBs followed by chromatin modifications in order to initiate damagesignaling proteins and repair mechanisms. These effects can be modulated by flavonoids which inhibit carcinogenesis process by multiple mechanisms including acting as antioxidants,induction of antioxidant defense and phase I/II enzymes, inhibition of DNA damaging proteins, stimulating repair mechanisms in normal cells and restoring genomic integrity in normalcells. Failure of repair mechanisms may result in the uncontrolled cell cycle, somatic mutations (chromosomal abnormalities, telomerase shortening etc.) and malignant tumorformation. Selected examples of flavonoids that are known to regulate carcinogenesis in normal cells: luteolin (1), apigenin (2), EGCG (3), quercetin (4), fisetin (5) and epicatechin (7).Abbreviations: P, phosphorylation; A, acetylation.
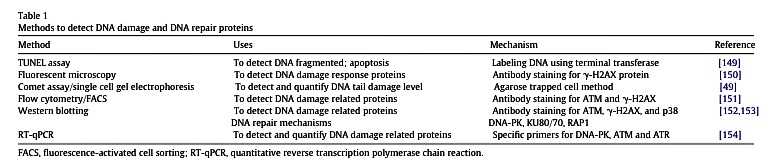
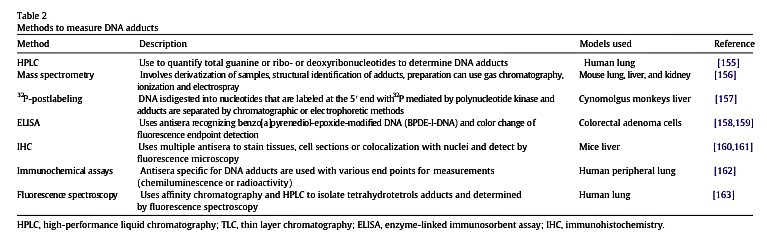
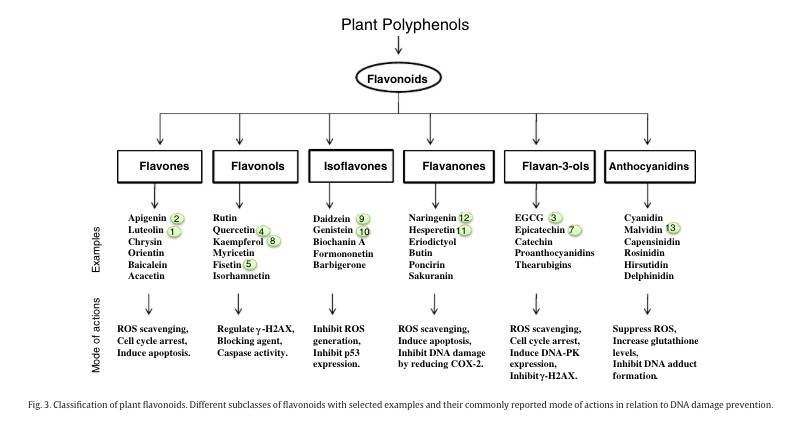
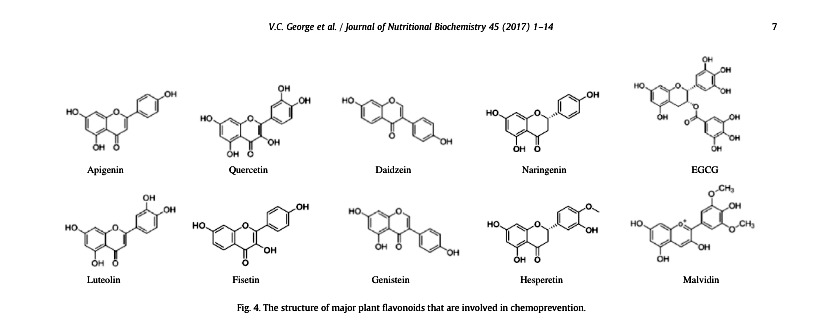
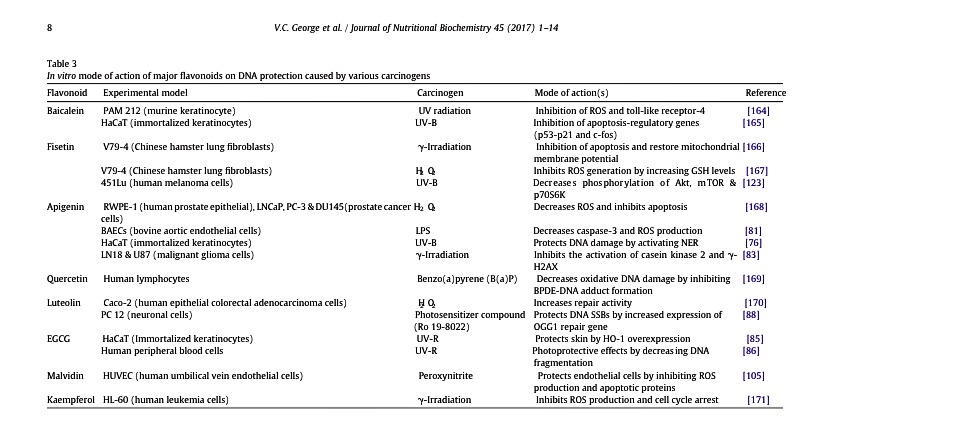
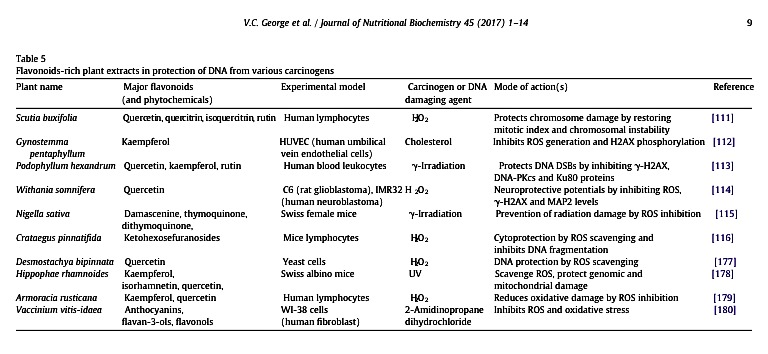
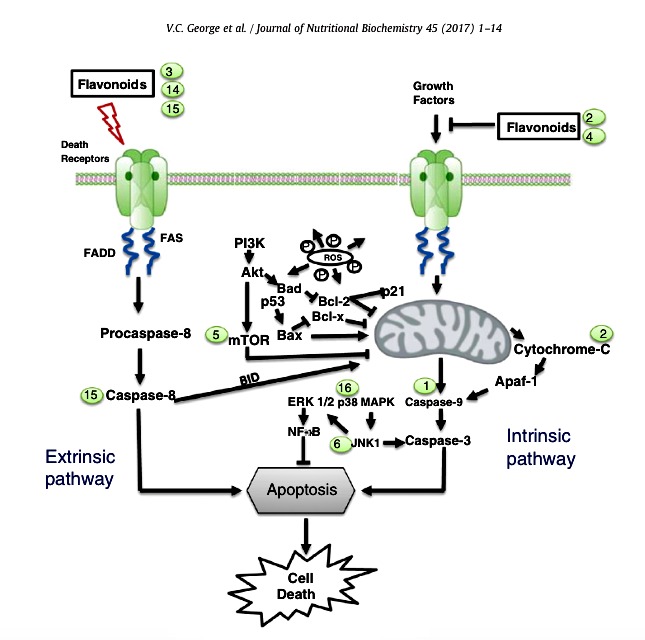
Fig. 5. Schematic illustration of flavonoids in apoptotic pathways in cancer cells. The different modes of action of flavonoids in extrinsic and intrinsic pathways which ultimately lead tocell death. Examples of flavonoid mediators: luteolin (1), apigenin (2), EGCG (3), quercetin (4), fisetin (5), chrysin (6), epicatechin (7), silibinin (14), EGC (15) and myricetin (16).
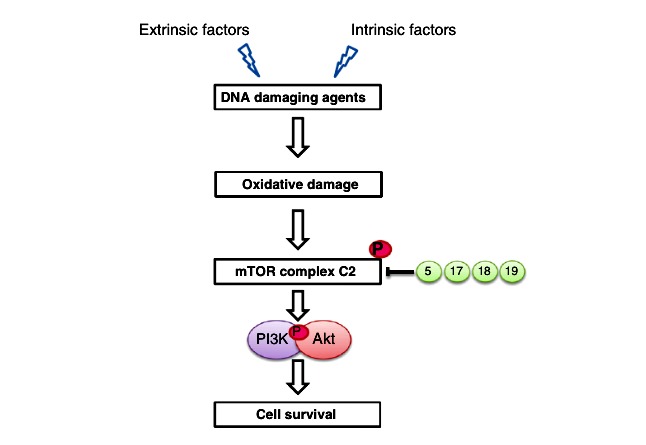
Fig. 6. Graphical representation of the influence of polyphenols in mTOR signaling in cancer cells. Expression levels of mTOR signaling activate continuous cell division and may lead tometastatic conditions. Flavonoids and other phytochemicals inhibit this process by dephosphorylation of mTOR complex formation associated with PI3K and Akt proteins, therebypromoting apoptotic cell death. Examples of polyphenol mediators: fisetin (5), piceatannol (17), caffeic acid (18) and butein (19).
- Citations1
- References194
-
- “Despite these phytochemical differences, H. speciosa and other Apocynaceae plants share some pharmacological activities—e.g., antidiabetic [49], antibacterial [50], cytotoxic [51], and anti-inflammatory [52] properties. The flavones luteolin (23) and apigenin (24)—reported for the first time in H. speciosa—and the flavonol quercetin (22) are some of the most efficacious plant flavonoids for cancer chemoprevention [53]. In general, plants rich in phenolic acids and flavonoids show a broad range of therapeutic properties that can contribute to a reduction in the incidence of chronic health problems, such as cancers, diabetes, and cardiovascular diseases [54][55][56]. “
Full-text (PDF)
Available from: H P Vasantha Rupasinghe, Dec 29, 2016 – Download full-text PDF
