
Published online 2009 Nov 3. doi: 10.1158/1535-7163.MCT-09-0448
Synthetic cannabinoid receptor agonists inhibit tumor growth and metastasis of breast cancer
Abstract
Cannabinoids have been reported to possess antitumorogenic activity. Not much is known, however, about the effects and mechanism of action of synthetic nonpsychotic cannabinoids on breast cancer growth and metastasis. We have shown that the cannabinoid receptors CB1 and CB2 are overexpressed in primary human breast tumors compared with normal breast tissue. We have also observed that the breast cancer cell lines MDA-MB231, MDA-MB231-luc, and MDA-MB468 express CB1 and CB2 receptors. Furthermore, we have shown that the CB2 synthetic agonist JWH-133 and the CB1 and CB2 agonist WIN-55,212-2 inhibit cell proliferation and migration under in vitro conditions. These results were confirmed in vivo in various mouse model systems. Mice treated with JWH-133 or WIN-55,212-2 showed a 40% to 50% reduction in tumor growth and a 65% to 80% reduction in lung metastasis. These effects were reversed by CB1 and CB2 antagonists AM 251 and SR144528, respectively, suggesting involvement of CB1 and CB2 receptors. In addition, the CB2 agonist JWH-133 was shown to delay and reduce mammary gland tumors in the polyoma middle T oncoprotein (PyMT) transgenic mouse model system. Upon further elucidation, we observed that JWH-133 and WIN-55,212-2 mediate the breast tumor-suppressive effects via a coordinated regulation of cyclooxygenase-2/ prostaglandin E2 signaling pathways and induction of apoptosis. These results indicate that CB1 and CB2 receptors could be used to develop novel therapeutic strategies against breast cancer growth and metastasis.
Introduction
Despite advances in the early detection of breast cancer, about 30% of patients with early stage have recurrent disease (1). Systemic treatment of breast cancer includes cytotoxic, hormonal, and immunotherapeutic agents, which are active at the beginning of therapy in 90% of primary breast cancers and 50% of metastases. After a variable period of time, however, progression occurs and multidrug resistance is observed (2–5). Thus, further studies are necessary to determine novel targets and mechanism-based agents with increased efficacy and low toxicity for prevention and treatment of this disease. In the present study, therefore, we analyzed the effects of synthetic cannabinoids on breast cancer cells.
Currently, there are three general types of cannabinoids: phytocannabinoids, and endogenous and synthetic cannabinoids. These function through two different specific cell surface G-protein coupled receptors, CB1 and CB2 (6, 7). The CB1 receptor is predominantly expressed in the central nervous system, whereas the CB2 receptor is expressed by immune cells. Cannabinoid receptors have been reported to be overexpressed in prostate, skin, and hepatocellular carcinoma (8–10). Experimental evidence has shown that cannabinoids inhibit the growth of tumor xenograft in mice (8, 11–14). Cannabinoids have been shown to inhibit tumor angiogenesis and directly induce apoptosis or cell cycle arrest in neoplastic cells (8, 11–14). Although these studies point to the potential application of cannabinoids as antitumor agents in various human cancer cells, not much is known about the molecular mechanism of cannabinoid-mediated antimetastatic and tumurogenic effects.
Cannabinoid receptors have also been shown to modulate several signaling pathways involved in the control of cell survival, including extracellular signal-regulated kinase (15), phosphoinositide 3-kinase (PI3K), p38 mitogen-activated protein kinase (MAPK; ref. 16), protein kinase B (Akt) signaling pathways and ceramide pathway (13, 17, 18) in various carcinomas. We have shown that synthetic cannabinoids also modulate the cyclooxygenase-2 (COX-2) signaling pathway in breast cancer cells.
Many human malignancies exhibit elevated prostaglandin levels due to upregulation of COX-2, a key enzyme in eicosanoid biosynthesis. COX-2 overexpression has been observed in about 40% of human breast carcinomas. COX-2 has been shown to modulate tumorogenesis by enhancing angiogenesis and resistance to apoptosis (19–21). Selective inhibitors of COX-2 have been shown to induce apoptosis in a variety of cancer cells, including those of the colon, stomach, prostate, and breast (22, 23). Recently, Gupta et al. showed that knocking down COX-2 along with EREG, MMP1, and MMP2 genes leads to abrogation of cancer growth (24). AP-1 has been suggested to play an important role in the regulation of the COX-2 expression in various cell lines (25). Classical regulation of cellular AP-1 activity occurs via two mechanisms: one is an increase in the transcription of c-fos and c-jun, and the other is the phosphorylation of c-Fos and c-Jun proteins (26). Recent studies also suggest a link between activation of GTPase proteins and COX-2 expression (27). Rho-related small GTPases are important in multiple cellular events, including actin cytoskeletal organization, cell proliferation and survival of cell and transcriptional regulations of genes. These small GTPases serve as molecular switches that cycle between a GTP-bound active form and a GDP-bound inactive form. Cdc42 is one of the important members of the Rho family responsible for tumor growth and progression.
In the present study, we analyzed the effects of synthetic nonpyschoactive cannabinoids on breast cancer growth and metastasis, and show that cannabinoid receptor agonists inhibit breast cancer cell growth and metastasis in vivo, including the polyoma middle T oncoprotein (PyMT) breast cancer transgenic mouse model. Furthermore, our signaling studies have revealed that synthetic cannabinoids modulate COX-2/prostaglandin E2 (PGE2) signaling pathways in breast cancer cells by inhibiting the activity of the downstream molecules c-Fos, c-Jun, and Cdc42. Thus, this study provides novel insight into the effects of synthetic nonpsychotic compounds on breast cancer growth and metastasis.
Materials and Methods
Reagents and Antibodies
Cell culture reagents were purchased from Gibco Laboratories. The following reagents were used in this study: anti-CB1 and anti-CB2 (Affinity Bioreagents); WIN-55,212-2, JWH-133, and AM 251 (Tocris Cookson); SR144528 (Sanofi Recherche); COX-2 (Caymen Chemical Co.); c-Fos, c-Jun (Cell Signaling); Cdc42 activation kit (Cytoskeleton); CD31 (BD Pharmingen); Ki67 (NeoMarkers); and terminal deoxynucleotidyl transferase–mediated dUTP nick end labeling (TUNEL) staining kit (Chemicon). MDA-MB231-luc-D3H2LN was purchased from Xenogen Laboratories.
Microarray Analysis
Total RNA was collected from JWH, WIN55, or vehicle-treated MDA-MB231 cells by using TRIzol reagent (Invitrogen), according to the manufacturer’s protocol. Microarray analysis was done at the Ohio State University Medical Center genomics core facility by using an Affymetrix Microarray gene chip containing 40,000 human genes.
Tissue Microarray Design
Paraffin-embedded, formalin-fixed specimens from 40 cases of breast carcinoma were identified from the archives of the Ohio State University Department of Pathology. In constructing the microarrays, 2-mm cores of tissues were taken, and 4-µm-thick sections were cut and placed on positively charged slides. Antigen retrieval was done by heat-induced epitope retrieval, where slides were placed in Dako TRS solution (pH 6.1) for 25 min at 94°C and cooled for 15 min. Slides were then placed on a Dako Autostainer, immunostaining system, for use with immunohistochemistry. Slides were incubated for 60 min in primary antibodies (1:200) and detected using a labeled polymer system, Imm-PRESS Anti-Rabbit Ig (peroxidase) Kit (Vector Laboratories) as per the manufacturer’s protocol. Staining was visualized with 3,3’-diaminobenzidine chromogen. Slides were then counterstained in Richard Allen hematoxylin, dehydrated through graded ethanol solutions, and coverslipped.
Cell Culture and Viability
MDA-MB231, MDA-MB468, and MB231-luc-D3H2LN cells were cultured in DMEM containing 10% heat-inactivated fetal bovine serum, 5 units/mL penicillin, and 5 mg/mL streptomycin. Cells were serum-starved and treated with JWH-133, WIN-55,212-2, or vehicle, respectively, for 24 h in a 37°C humidified chamber. Cell viability was determined by the MTTassay as per the manufacturer’s instructions (Chemicon).
Western Blot Analysis
Western blot analysis was done as described previously (28).
Quantification of Apoptosis and Cell Cycle Analysis by Flow Cytometry
MDA-MB-231 cells were grown at a density of 1 × 106 in 100-mm culture dishes and were treated with JWH-133 and WIN-55,212-2 (10 µmol/L) or vehicle alone for 24 h. For COX-2 inhibitor experiment, cells were treated with 10 µmol/L NS-398 (Caymen Chemical) with or without JWH-133, WIN-55,212-2 (10 µmol/L), or vehicle for 24 h. The cells were processed for labeling with fluorescein-tagged deoxyuridine triphosphate nucleotide and propidium iodide by use of Apo-direct apoptosis kit (Phoenix Flow Systems) as per the manufacturer’s protocol. The labeled cells were analyzed by FACS (BD Bioscience). Results were analyzed using ModFit LT software (Verity Software House) for cell cycle and apoptosis.
Confocal Microscopy
Confocal microscopy was done as described (28).
CB2 Small Interfering RNA
MDA-MB231 cells were transfected with specific CB2 targeting small interfering RNA (siRNA) designed to knock down human CB2 expression (Dharmacon). Briefly, MDA-MB231 cells (70% confluent) were transfected with 100 pmol CB2 siRNA or nontargeting control using Lipofect AMINE 2000 (Invitrogen) according to the manufacturer’s protocol. After 24 h, cells were treated overnight with vehicle, JWH-133, or WIN-55,212-2 (10 µmol/L) and subjected to migration assay. The cells were evaluated by Western blot for the expression of CB2 using anti-CB2 antibodies.
Chemotactic Assays
Migration assays were done as described previously (29).
Animal Studies
The in vivo experiments were carried out in compliance with the guidelines and protocols approved by Institutional Animal Care and Use Committee of Beth Israel Deaconess Medical Center and The Ohio State University. Severe combined immunodeficient CB-17 mice (Charles River Laboratories Inc.), 4 to 6 wk old, were used for all xenograft studies. For lung metastasis, 1 × 106 (100 µL) viable MDA-MB231-luc-D3H2LN cells were injected into the lateral tail vein. After 24 h the mice were treated i.p. with JWH-133, JWH+SR, WIN-55,212-2, WIN+AM, WIN+SR, WIN+AM+SR (5 mg/ kg/d), or vehicle (n = 6) in 100 µL of PBS for 4 wk. End point assays were conducted at 4 wk after injection.
Bioluminescent Imaging and Analysis
The mice were assessed weekly using whole-body bioluminescent imaging to quantify relative amounts of tumor burden (IVIS System 200, Xenogen Corporation). The mice were anesthetized and injected i.p. with 1.5 mg of D-luciferin in 0.1 mL PBS. Bioluminescent images were collected between 2 and 5 min after injection. The levels of light emitted from the bioluminescent tumor cells were detected by the IVIS camera system, integrated, digitalized, and displayed. For bioluminescent imaging plots, photon flux was calculated for each mouse by using region of interest encompassing the lungs of the mouse.
Subcutaneous Xenografts
Tumors were induced by s.c. flank injection of 3 × 106 (150 µL) MDA-MB231 cells in PBS in severe combined immunodeficient CB-17 mice. When tumors were palpable, the animals were assigned randomly to various groups (n = 6) and injected peritumorally with JWH-133, JWH+SR, WIN-55,212-2, WIN+AM, WIN+SR, WIN+AM+SR (5 mg/kg/d), or vehicle for 4 wk. Tumors were measured with external calipers and volume was calculated as volume = length × (width) 2 × 0.52 mm3.
Transgenic Mice
Male PyMT mice on a C3H/B6 × FVB-C3H/B6 background were randomly bred with wild-type C3H/B6 females to obtain female mice heterozygous for the PyMT transgene. All littermates were weaned and tail-clipped at age 4 wk, genotyped (30), and distributed randomly into the treatment groups. Female hetrozygotes were used in this study. After weaning, the animals were both weighed and examined for mammary gland tumors every week. The mice were injected i.p. with JWH-133 (5 mg/kg/d) or vehicle daily. After 8 wk, the mice were sacrificed and tumors were dissected, weighed, and divided into pieces, which were cryoembedded in cryo-preservative O.C.T compound (Tissue-Tek) for further analysis.
Cdc42 Activation
Serum starved MDA-MB231 cells were treated with 10 µmol/L of JWH-133, WIN-55,212-2, or vehicle. Cdc42-GTP was pulled down from lysates (2,000 mg) of treated cells using Cdc42 Activation kit (Cytoskeleton) according to the manufacturer’s protocol.
Extraction of Nuclear and Cytoplasmic Fractions
Nuclear and cytoplasmic fractions were extracted as described (28).
Immunostaining and TUNEL
Samples from tumor xenografts were dissected and embedded in ornithine carbamyl transferase. Standard immunohistochemistry techniques were used as per the manufacturer’s recommendations (Vector Laboratories) using the primary antibody against Ki 67 (Neomarkers) at a dilution of 1:100 and CD31 (BD Pharmingen) at a 1:50 dilution for 60 min at room temperature. Vectastain Elite ABC reagents (Vector Laboratories) using avidin DH: biotinylated horseradish peroxidase H complex with 3,3’-diaminobenzidine (Polysciences) and Mayer’s hematoxylin (Fisher Scientific) were used for detection of the bound antibodies. The nuclei for Ki67 staining and CD31 expression in vessels were quantitated in 5 random microscopic (×10) fields per tumor. The results were expressed as the percent change with vehicle-treated samples normalized to 100%.
To study in vivo apoptosis, TUNEL was done using in situ cell death detection kit (Chemicon) on the xenograft tumors. Cells were visualized by fluorescence microscopy and images were acquired using Leica Axiovert S100 TV microscope (Carl Zeiss). Cells stained positive for TUNEL were scored, and induction of apoptosis determined by comparison with vehicle control.
Reverse Transcriptase and Real-time PCR
Total RNA was extracted from cell lines and frozen tissues by Trizol reagent (Invitrogen). Reverse transcriptase PCR (RT-PCR) reaction was done using RT-PCR kit (Clontech, CA) using sense primer CB1-F (5′-GTGTCTTTGGGAA-GATGAACAAGC-3′) and the antisense primer CB1-R (5′-GACGTGTCTGTGGACACAGACATGG-3′) to amplify the CB1 receptor. For CB2 receptor, sense primer 5′ CCATG-GAGGAATGCTGGGTG 3′ and antisense primer 5′ TCAG-CAATCAGAGAGGTCTAG 3′ were used. PCR reactions were done for 30 s at 93°C, 1 min at 59°C, and 1 min at 69°C for 28 cycles. Real-time PCR was done for COX-2 expression with GAPDH as endogenous control. TaqMan analysis and subsequent calculations were done with an ABI Prism 1 7700 Sequence Detection System (PerkinElmer). For each sample, 100 ng of total RNA were subjected to realtime PCR according to the protocol provided by the manufacturer of the TaqMan Master Mix Reagents Kit (ABI). The sequences of the forward, reverse, and double-labeled oligonucleotides for COX-2 were as follows: forward, 5′-AAG ATG CGC AAC CTG GAG AC-3′; reverse, 50-CCT TCT TCT GCA GCC GCT C-30; double-labeled oligonucleotide, 50-FAM-CAC AAG GTG CTG GAG CTG ACG GGC-TAM-RA-30. Amplification of GAPDH was done in the same reaction tubes as an internal standard with an alternatively labeled probe (VIC labeled probe), and the results were normalized to GAPDH mRNA levels. Assays were done in triplicate for each standard and treated sample.
Measurement of PGE2 Protein
Serum-starved MDA-MB-231 cells were then treated with 10 µm each of JWH-133, WIN-55,212-2, or vehicle for 18 h. PGE2 protein levels in the supernatants were assayed using a PGE2 EIA Kit (Caymen Chemicals) following the manufacturer’s instruction. Optical density was measured at 420 nm. PGE2concentration was calculated by comparing the data with the known standards for PGE2 proteins.
Statistical Analysis
Student’s two-tailed t test was used to compare vehicle and cannabinoid-treated groups. P < 0.05 was considered to be statistically significant. For all graphs, * indicates P < 0.05; ** indicates P < 0.01.
Results
Cannabinoid Receptors Are Expressed in Human Breast Cancer Cells
Cannabinoids exert their effect through two G-protein coupled receptors, CB1 and CB2. To elucidate the role of cannabinoid receptors in breast cancer, we assessed their expression on 40 primary human breast tumors using tissue microarray analysis and observed 28% CB1 and 35% CB2 receptor staining. However, low levels of CB1 (3%) and CB2 (5%) expression were observed in 10 normal epithelium samples (Fig. 1A). The expression of these receptors was also assessed in MDA-MB231, MDA-MB231-luc-D3H2LN, and MDA-MB468 breast cancer cell lines by mRNA level by RT-PCR (Fig. 1B) and at protein level by Western blot analysis (Fig. 1C). We further confirmed the expression of these receptors in these cell lines by confocal microscopy (Fig. 1D). We observed that expression of the CB1 receptor was less than that of the CB2 receptor in these cell lines. Taken together, these results suggest that both CB1 and CB2 receptors are expressed in primary breast cancer tissues, as well as breast cancer cell lines.
Synthetic Cannabinoids Inhibit Cell Growth and Migration in Breast Cancer Cells
Although recent studies have shown that cannabinoids have an inhibitory effect on the cell growth and migration of cancer cells (10, 29), not much is known about the effects of nonpsychoactive synthetic cannabinoids on breast cancer growth and progression. Therefore, we investigated the effects of the synthetic CB1 receptor agonist JWH-133 and CB1/CB2 receptor agonist WIN-55,212-2 on breast cancer growth and migration. We observed a dose-dependent inhibitory effect of JWH-133 and WIN-55,212-2 on proliferation of MDA-MB231 and MDA-MB468 cells lines. Maximum inhibition of 58% and 42% was observed with 10 µmol/L JWH-133 and WIN-55,212-2, respectively, in MDA-MB231 cells, compared with vehicle-treated cells (Fig. 2A, left). Similarly, a significant inhibition of 48% and 45% was observed with 10 µmol/L JWH-133 and WIN-55,212-2, respectively, in MDA- MB468 cells compared with the vehicle-treated cells (Fig. 2A, right).

Further, we investigated the effects of these cannabinoids on migration of breast cancer cells and observed a significant dose-dependent inhibition in migration. Maximum inhibition of 42% and 47% was observed in MD-MB231 with JWH-133 and WIN-55,212-2 at 10 µmol/L concentration compared with vehicle-treated cells (Fig. 2B, left). Similar results were obtained with MDA-MB468 cells showing an inhibition of 45% and 40%, respectively, with 10 µmol/L JWH-133 and WIN-55,212-2 treatment compared with the control-treated cells (Fig. 2B, right). There was no adverse effect on the viability of cells using different concentrations of cannabinoids in this study, as confirmed by trypan blue staining. Taken together, these data suggest that cannabinoids inhibit cell viability and cell migration in breast cancer cell lines.
CB2 Specific siRNA Blocked Effect of Cannabinoid Treatment
To confirm that these effects are cannabinoid receptor-mediated, we used specific human CB2 targeting siRNA. MDA-MB231 cells, transfected with CB2 siRNA (100 pmol), were treated overnight with vehicle, JWH-133, or WIN-55,212-2 (10 µmol/L). CB2 siRNA was able to block JWH-133– and WIN-55,212-2–mediated migration towards 10% serum (Fig. 2C, left). In addition, to evaluate the knockdown efficiency, we did Western blot using anti-CB2 antibody and found a significantly decreased level of CB2 expression in siRNA-transfected cells (Fig. 2C, right). These data indicate that the effects of JWH-133 and WIN-55,212,2 are mediated through CB2 receptor.
Synthetic Cannabinoid Treatments Lead to Reduced Tumor Burden, Proliferation, and Angiogenesis In vivo
We next investigated the effect of these synthetic cannabinoids on tumor growth in vivo. Tumors induced in immunodeficient mice by s.c. flank injection of MDA-MB231 cells were reduced significantly on treatment with agonists JWH-133 (46%; Fig. 3A) and WIN-55,212-2 (42%; Fig. 3B) compared with vehicle-treated mice. Specific cannabinoid receptor antagonists were used to observe if the effects were receptor mediated. A significant reversal of ~65% to 75% inhibition of tumor growth was observed when mice were treated either with CB2-specific antagonist (SR144528) along with JWH-133, or with CB1/CB2 antagonist (AM 251 or SR144528) along with WIN-55,212-2, compared with the control-treated group. Furthermore, a significant 85% reversal of effect on tumor burden was observed when a combination of CB1/CB2 antagonists (AM251 and SR144528) was used along with WIN-55,212-2 (Fig. 3C). These data indicate that synthetic cannabinoids mediate tumor suppressive effects through their receptors CB1 and CB2.

For a tumor to grow beyond a minimal size, it must generate a new vascular supply. Thus, blocking the angiogenic process constitutes one of the most promising antitumor approaches now available. To quantify the angiogenesis and proliferation in treated tumors, the xenograft tumors from mice were subjected to immunohistochemical analysis for vascularization and cell proliferation markers. We observed a significant decrease of 43% and 40% in CD31 (an endothelial cell-specific antigen marker) in JWH-133– and WIN-55,212-2–treated tumors, respectively. Further, control carcinomas showed long and branched blood vessels, whereas vessels in JWH-133– and WIN-55,212-2–treated tumors were narrow. Similarly, Ki67 staining (marker for proliferation) showed a significant 39% and 38% reduction in tumors treated with JWH-133 and WIN-55,212-2, respectively, relative to control tumors (Fig. 3D). These results suggest that synthetic cannabinoids inhibit angiogenesis and cell proliferation, and these effects were mediated by CB1/CB2 receptors in tumors.
Synthetic Cannabinoid Receptor Agonists Inhibit Metastasis of MDA-MB 231 Cells to Lungs In vivo
Because we showed that synthetic cannabinoids have a significant inhibitory effect on the growth and migration of MDA-MB231 breast cancer cells in vitro, we next studied the effect of these cannabinoids on metastasis in vivo. For this, MDA-MB231-luc-D3H2LN, which are luciferase-positive cells, were injected i.v. in immunocompromised mice. Metastatic activity was assayed by bioluminescent imaging of luciferase-transduced cells every week. We observed a 76% and 67% reduction in lung metastasis in JWH-133– and WIN-55,212-2–treated mice, respectively, relative to the control group. Furthermore, to determine if the effects were mediated through CB1 and CB2 receptors, we treated mice with CB1- and CB2-specific antagonists. Inhibition in lung metastasis upon synthetic cannabinoids treatment was reversed significantly in antagonist-treated mice (Fig. 4A – C). We further analyzed the surface nodules in lungs in these mice and observed that it correlated well with the bioluminescence data. We observed a 75% and 60% reduction in lung nodules in JWH-133– and WIN-55,212-2–treated mice, respectively, compared with the control mice, and these effects were abrogated in the presence of CB1- and CB2-specific antagonists. Interestingly, the effect was almost completely reversed (97%) when both antagonists, AM 251 and SR144528, were used with WIN-55,212-2 (Fig. 4D). These data suggest that both of the synthetic cannabinoids possess antimetastatic properties that are mediated through their specific receptors.
Synthetic Cannabinoids Retard the Growth of Mammary Gland Tumors in PyMT Transgenic Mouse Model
PyMT transgenic mice share many features of human breast cancer, and multiple stages of tumor progression are recapitulated. Hence, the effect of synthetic cannabinoids on the spontaneous mammary gland carcinogenesis was studied using the PyMT transgenic model. Importantly, we observed a delay of approximately 1 week in posterior and anterior mammary gland tumor formation in the JWH-133–treated mice compared with the control group. Furthermore, our results also revealed that PyMT mammary tumors were greatly reduced in size in the JWH-133–treated group, relative to the control group. The average tumor mass in JWH-133–treated mice was 0.12 g compared with 0.28 g in vehicle-treated PyMT mice (Fig. 5A and B). In addition, we showed that JWH-133–treated tumors showed a significant decrease of 40% and 37% of Ki67 (Fig. 5C) and CD31 (Fig. 5D) staining, respectively, compared with vehicle-treated group. Taken together these data suggest that cannabinoids increased tumor latency, and decreased tumor growth and aggressiveness in PyMT mice.
Microarray Analysis
To investigate the mechanisms involved in the cannabinoid-mediated tumor-suppressive effects, we carried out microarray analysis by using a gene chip containing 40,000 human genes. Among the various genes that were down-regulated in JWH-133 and WIN-55,212-2 cells compared with control vehicle-treated cells, we selected the COX-2/ PGE2 signaling pathway for our further investigation and then confirmed these results by using Western blot analysis.
Synthetic Cannabinoids Modulate COX-2/PGE2 Signaling in Breast Cancer
To elucidate the molecular mechanism of synthetic cannabinoid-mediated inhibition of tumor growth and metastasis, we analyzed the effects of these synthetic cannabinoids on the COX-2/PGE2 pathway. COX-2 is responsible for high levels of prostaglandin production during inflammation and cancer growth and metastasis (31).We measured PGE2 levels in supernatants of MDA-MB231 cells treated with vehicle, JWH-133, or WIN55, 212-2 by ELISA. We observed a significant decrease in cannabinoid-treated cells compared with vehicle-treated cells. The results are mean ± S.E. of three independent experiments (Fig. 6A). Next, we observed a significant decrease in the expression of COX-2 in JWH-133– and WIN-55,212-2–treated MDA-MB231 cells (Fig. 6B, left). Further, we observed an 8-fold and a 6-fold decrease in the expression of COX-2 in tumors excised from JWH-133– and WIN-55,212-2–treated mice, respectively, relative to control tumors (Fig. 6B, right). The decrease in COX-2 expression in these tumors was further confirmed by Western blot analysis (Fig. 6B, inset).
Synthetic Cannabinoids Modulate COX-2 Regulators in Breast Cancer
Recently, regulation of COX-2 expression by small GTPases has been reported in different cell lines. Hence, we also investigated the effect of synthetic cannabinoids on the activity of Cdc42, which is an important member of the GTPase family. We observed a significantly low activity of Cdc42 in JWH-133– and WIN-55,212-2–treated MDA-MB231 cells compared with vehicle-treated cells (Fig. 6C, left). Because c-Fos and c-Jun have been shown to interact with COX-2 promoter transcriptionally to activate COX-2 expression (32), we further investigated the effect of synthetic cannabinoids on these transcription factors. Nuclear extracts of MDA-MB231 cells obtained from cannabinoid-treated cells showed reduced expression of c-Fos and c-Jun compared with vehicle control cells (Fig. 6C, middle). These data suggest that the inhibition of COX-2 expression by synthetic cannabinoids may be regulated by reduced expression of transcription factors c-Fos and c-Jun in breast cancer cells.
Synthetic Cannabinoids Arrest Cell Cycle and Induce Apoptosis in MDA-MB231 Cells In vitro and in Tumor Samples
Overexpression of COX-2 has been reported in a number of carcinomas. Its overexpression has been associated with uncontrolled cell proliferation and inhibition of apoptosis. Hence, to see whether the growth inhibitory effects of cannabinoids were due to cell cycle arrest and apoptosis, MDA-MB231 cells were treated with JWH-133 and WIN-55,212-2 (10 µmol/L) or vehicle for 24 hours, and the effect was determined using fluorescein-tagged deoxyuridine triphosphate nucleotide and propidium iodide. We observed significant cell cycle arrest and apoptosis in cells treated with cannabinoids for 24 hours compared with control. More specifically, the cells were arrested in the G0-G1 phase when treated with JWH-133 (69.89 ± 1.25) and WIN-55,212-2 (62.33 ± 1.88), as compared with the control (54.17 ± 3.6). The induction of apoptosis was negligible in vehicle control cells (3.47 ± 4.8) relative to JWH-133–treated cells (30.61 ± 0.94) and WIN-55,212-2–treated cells (22.53 ± 1.14%; Fig. 6, top). To validate the in vitro results, we also analyzed tumors derived from mice for apoptosis. We observed a significant induction of apoptosis of 40% and 20% in JWH-133– and WIN-55,212-2–treated tumors relative to vehicle-treated control tumors (Fig. 6, left). Further, to confirm that the induction of apoptosis is a direct consequence of downregulation of COX-2 by synthetic cannabinoids, we used COX-2 selective inhibitor NS-398 in MDA-MB231 cells. NS-398 has been shown to inhibit proliferation and induce apoptosis in various carcinomas (23). We observed a significant induction of apoptosis when these cells were treated with the inhibitor along with JWH-133 or WIN-55,212-2 compared with the treated vehicle control (Fig. 6, right). Taken together, these results suggest that the synthetic cannabinoids may block tumor growth by inducing cell cycle arrest and apoptosis in human breast cancer tumors.
Discussion
Breast cancer is the most common cancer and the second leading cause of cancer death in American women. Despite recent advances in early detection, mortality still remains very high due to the emergence of refractory tumors associated with multidrug resistance. Therefore, additional alterative therapies with increased efficacy and low toxicity should be explored. Although studies have recently suggested that cannabinoids exert potential antitumor effects on a wide spectrum of human tumor cell lines in culture and in xenograft studies (8, 10, 14, 18, 32–41), not much is known about the effects of nonpsychoactive synthetic cannabinoids on breast cancer progression and metastasis. In the present study, we investigated the antitumoral and metastatic properties of two synthetic cannabinoids (JWH-133 and WIN-55,212-2) against breast cancer cell lines. Here we report for the first time that synthetic cannabinoids significantly inhibit tumor growth and metastasis in vitro and in vivo in different mouse model systems, including PyMT breast cancer transgenic mouse model systems. Furthermore, we have shown that these effects are modulated through the COX-2/PGE2 signaling pathway.
Synthetic cannabinoids exert a wide variety of biological effects by mimicking endogenous compounds (endocannabinoids), which exert their effects through two cannabinoid-specific G-protein coupled receptors, CB1 and CB2. In this study we have shown for the first time overexpression of cannabinoid receptors CB1 and CB2 in primary human breast tumor samples by tissue microarray analysis. The expression of these receptors was higher in poorly and well-differentiated breast cancer compared with ductal carcinoma in situ tumors and normal breast tissue. We also observed expression of these receptors in breast cancer cell lines MDA-MB231 and MDA-MB468. Previously, both CB1 and CB2 receptors were reported to be overexpressed in various cancers, such as prostate, skin, and hepatocellular carcinoma (8–10).
We observed that both synthetic cannabinoid JWH-133, which specifically binds to CB2 receptors, and WIN-55,212-2, which binds to CB1 and CB2 receptors, significantly inhibit cell proliferation and migration of breast cancer cell lines in vitro. The inhibition of migration of MDA-MB231 cells by JWH-133 and WIN55-212-2 was blocked by knockdown of CB2, indicating that these effects are mediated through CB2 receptor. Although endocannabinoid (R)-methanandamide has been shown to inhibit growth and metastasis of breast cancer cells in vivo, here we show that synthetic cannabinoids that do not possess psychoactivity also significantly inhibit tumor burden and lung metastasis in multiple in vivo mouse models. We have for the first time shown that JWH-133 treatment delays and significantly reduces mammary tumors in the PyMT transgenic mouse model system. This mouse model has been used to study the effects of antitumor drugs as it recapitulates the processes found in human breast cancer progression and spontaneous metastasis (40, 41). Contrary to these findings, however, one report by McKallip et al. has shown that D (9)-tetrahydrocannabinol enhanced breast cancer growth and metastasis specifically in cells expressing low levels of cannabinoid receptors by suppressing the antitumor immune response (42), suggesting that cannabinoid exposure may increase the incidence of breast cancer, as well as other cancers that do not express cannabinoid receptors. Previous in vitro and in vivo studies have shown that endocannabinoids and synthetic cannabinoids have antitumor effects in gliomas, prostate, lung, and colon cancer (8, 9, 11, 29, 43, 44). The in vivo inhibitory effects of JWH-133 and WIN-55,212-2 in tumor growth and metastasis were reversed by CB2 and CB1 antagonists, indicating that both of these receptors regulate tumor growth and metastasis in vivo.
The molecular mechanism of cannabinoid-mediated inhibition of tumor growth and metastasis is not well-known. Recently, it has been shown that Δ9-tetrahydrocannabinol can induce apoptosis in colorectal cancer cells by inhibiting the RAS-MAPK/ERK and P13K-AKT cell survival pathways and the increased expression of the proapoptotic protein BAD (45). In addition, it has also been reported that (R)-methanandamide-treated cells show decreased phosphorylation of focal adhesion-associated protein kinase and Src (46). In our study, we have shown that synthetic cannabinoids significantly suppress expression of proangiogenetic gene COX-2. These effects were observed in MDA-MB231 cells and cannabinoid-treated tumor samples. Furthermore, we observed a significant decrease in PGE2 secretion in cannabinoid-treated MDA-MB231 cells. This study is in contrast to previous studies where endocannabinoids have been shown to increase COX-2 expression, and tumor growth in lung cancer and cerebral microvascular endothelium (31, 47). This discrepancy could be explained as anandamide has also been shown to mediate its effects through a receptor-independent pathway that may lead to upregulation of COX-2 pathway. Recent data have shown that COX-2–derived prostaglandins modulated the production of proangiogenetic factors in colon cancer cells, and that COX-2 overexpression could result in resistance to apoptosis, increased invasiveness, and tumor angiogenesis (31, 47, 48). We show that synthetic cannabinoid treatment leads to apoptosis in tumors and arrest of MDA-MB231 cells in G0-G1 phase. Furthermore, to confirm a direct relationship of down modulation of COX-2 and induction of apoptosis by synthetic cannabinoids, we used NS-398, which is one of the COX-2 inhibitors and has been shown to induce apoptosis (23). We found a significant induction of apoptosis in cells treated with NS-398 and JWH-133 or WIN55,212-2 compared with the vehicle-treated control. We also observed significant reduction in angiogenesis (CD31 staining) and proliferation (Ki67 staining) in tumors derived from mice treated with cannabinoids. COX-2 has been shown to regulate activity of GTPases and transcription factors (25, 27). In our present study, we observed reduced Cdc42 activity and nuclear expression of transcription factors c-Jun and c-Fos in MDA-MB231 cells. These studies, therefore, suggest that cannabinoid regulation of COX-2 pathways may lead to reduced angiogenesis and subsequent apoptosis in breast cancer. It may be one of the mechanisms by which cannabinoids modulate tumor growth and metastasis. Thus, cannabinoids along with COX-2 inhibitors may represent novel chemopreventive tools for the treatment of breast cancer.
Acknowledgments
We are thankful to Drs. Wenle Wang and Sabrina Simpson, Department of Pathology, The Ohio State University, Columbus, Ohio for analyzing the tissue microarray analysis and immunohistochemical slides, and to Susie Jones, Department of Pathology, The Ohio State University, Columbus, Ohio for the immunostaining of TMA and tumor slides.
Grant support: NIH Grant CA109527 to R.K. Ganju.
Footnotes
Disclosure of Potential Conflicts of Interest
No potential conflicts of interest were disclosed.
References
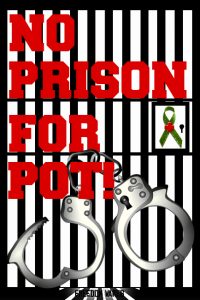
