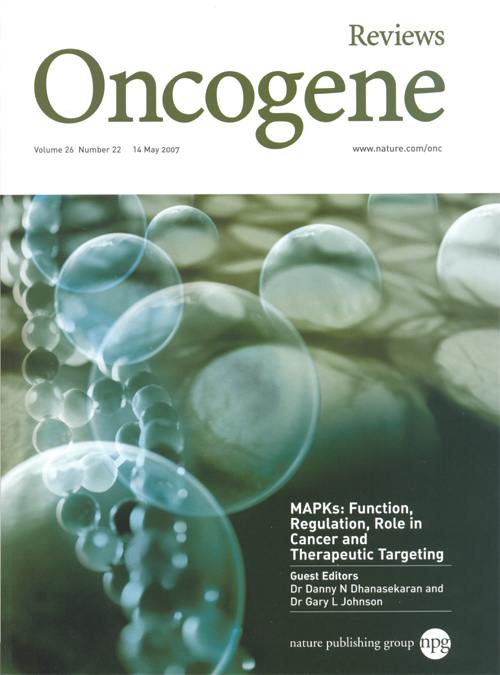
www.nature.com/onc
ORIGINAL ARTICLE
The orphan receptor GPR55 drives skin carcinogenesis
and is upregulated in human squamous cell carcinomas
E Pe ́rez-Go ́mez1, C Andradas1, JM Flores2, M Quintanilla3, JM Paramio4, M Guzma ́n1,5 and C Sa ́nchez1
G protein-coupled receptors (GPCRs) are the direct or indirect target of 450% of current therapeutic drugs. In the context of oncology, however, despite the increasing evidence showing a link between GPCR deregulation and cancer, just a few GPCRs are being exploited as targets of chemotherapeutic agents. Indirect lines of evidence suggest that the orphan GPCR GPR55 may have a role in cancer physiopathology. Thus, increased levels of the phospholipid lysophosphatidylinositol (LPI), a putative GPR55 endogenous ligand,1 have been found in plasma and ascites from patients with ovarian cancer compared with healthy women or with women with non-cancerous pathologies.2,3 In addition, epithelial cells4 and fibroblasts5 are able to generate mitogenic LPI upon ras-driven transformation. More recently, it has been shown that GPR55 modulates cancer cell migration6 and proliferation7,8 in vitro, and tumor growth in a xenograft-based model of glioblastoma.7 However, the actual role of GPR55 in malignant transformation in vivo remains unknown. One of the best established paradigms for studying the mechanisms underlying this process is the mouse skin model of two-stage carcinogenesis.9 Animals subjected to this experimental protocol evolve through different stages of cancer progression: first, in the ‘initiation’ phase, key genes are mutated in keratinocyte stem cells by topical exposure to the mutagenic agent 7,12- dimethylbenz[a]anthracene (DMBA).9 After the initiation stage, the population of mutated cells expands as a result of the repeated topical application of a proliferation-inducing agent such as the phorbol ester 12-O-tetradecanoylphorbol-13-acetate (TPA), UV radiation or wounding. During this ‘promotion’ stage, animals develop benign hyperplastic skin lesions termed papillomas.9 With variable frequency these structures may progress to malignant invasive squamous cell carcinomas or the more aggressive spindle cell carcinomas. This ‘progression’ stage occurs independently of tumor promoters and is the consequence of the genetic alterations accumulated during the proliferation of initiated cells.9 Here, by using this experimental protocol, as well as other in vivo and in vitro approaches, we demonstrate that GPR55 has an essential role in skin tumor development.
GPR55 is expressed in mouse epithelia
First, we analyzed the expression of GPR55 in different mouse- stratified epithelia. As shown in Supplementary Figure 1, the receptor was moderately expressed in mouse skin, specifically in the epidermis (Supplementary Figure 1A). A similar pattern of GPR55 expression was found in other mouse-stratified epithelia. Thus, a discreet GPR55 immunoreactivity was detected in the epithelial compartments of the oral cavity (Supplementary Figure 1B), esophagus (Supplementary Figure 1C) and stomach (Supplementary Figure 1D). Interestingly, GPR55-positive cells were mainly located in the most proliferative layers of those epithelia (Supplementary Figure 1). Controls of the specificity of GPR55 staining are provided in Supplementary Figure 2.
E Pe ́rez-Go ́mez et al
Figure 2. GPR55 mediates TPA-induced epidermal hyperproliferation. WT and GPR55 KO mice were subjected to a single skin topical application of TPA. The following features were analyzed at the indicated times: skin architecture (a) and epidermal thickness (b) by H&E staining; the levels of different markers of cell proliferation by western blot (c) and semi-quantitative PCR (d); and GPR55 levels in WT mouse skin by RTQ–PCR (e). **Po0.01 vs t0 (time of TPA application); ##Po0.01 vs the corresponding group of WT mice. Scale bar, 200 mm.
Exacerbated proliferation of initiated cells is crucial in the first stages of tumor development. Consequently, we analyzed whether GPR55 expression confers a proliferative advantage on epidermal cells in vivo in response to a promoting agent. We treated the skin of GPR55-deficient mice and their corresponding WT littermates topically with a single dose of TPA. No significant differences were found in the skin architecture of WT and GPR55 KO mice before TPA treatment (Figure 2a, upper panels). However, TPA challenge induced a remarkable increase in epidermal thickness in WT animals that was significantly attenuated in GPR55 KO mice (Figures 2a and b). Known drivers of cell proliferation in this model (phosphorylated ERK, c-Fos and Cyclin D1) were increased upon TPA treatment in WT mice, an effect that was not observed in the corresponding GPR55 KO animals (Figures 2c and d). Interestingly, TPA-induced hyperplasia in the WT population was accompanied by a strong upregulation of GPR55 levels in the skin (Figures 2d and e and Supplementary Figure 3). Proliferating cell nuclear antigen staining revealed that, after TPA challenge, the skin of GPR55-deficient mice presented significantly less proliferating cells than the skin of WT animals (Figure 3).
It is well described that, together with epidermal hyperproli- feration, TPA topical application on mouse skin induces local inflammation,11 which can be detected by the increase in dermal celullarity (Figure 2a). In agreement with this notion, we observed an increased number of inflammatory (CD45-positive) cells infiltrating the dermis (Supplementary Figures 4A and B), and augmented levels of ILb1 and TNFa (Supplementary Figures 4C and D). Importantly, we did not observe these characteristics in GPR55 KO mice (Figure 2 and Supplementary Figure 4).
Figure 3. GPR55-deficient mice present reduced epidermal proliferation in response to TPA. (a) Immunofluorescence analysis of proliferating (that is, proliferating cell nuclear antigen (PCNA)-positive) cells in the skin of WT and GPR55 KO mice treated topically with a single TPA application. PCNA is stained in pink and cell nuclei in blue. (b) Quantification of PCNA-positive cells. **Po0.01 vs t0 (time of TPA application); ##Po0.01 vs the corresponding group of WT mice. Scale bar, 200 mm.
E Pe ́rez-Go ́mez et al
Figure 4. Cells undergoing proliferation express GPR55. (a) WT mice were topically treated with a single dose of TPA for 48 h, and GPR55 (red) and proliferating cell nuclear antigen (PCNA, green) were analyzed by immunofluorescence. (b and c) GPR55 and PCNA protein expression was studied in a representative papilloma (b) and a representative carcinoma (c), generated in a WT mouse topically treated with a single dose of DMBA followed by the repeated application of TPA for 22 weeks. Cell nuclei are stained in blue. Scale bars, 100 mm.
GPR55 enhances the tumorigenic properties of skin cancer cells
To further demonstrate the functional relevance of GPR55 in skin tumorigenesis, we performed a series of in vitro and in vivo experiments with PDV skin carcinoma cells,12 which express GPR55 (Figure 5a). GPR55 expression was stably knocked down in these cells by means of selective shRNAs (Figure 5a). First, we observed that receptor silencing decreased the number of viable cells (Figure 5b). Additionally, anchorage-independent growth was impaired by GPR55 knockdown. Thus, GPR55-silenced cells generated significantly less and smaller colonies in soft agar than the corresponding control shRNA-transfected cells (Figure 5c). Invasion through Matrigel was also hampered in GPR55-deficient cells (Figure 5d). To further demonstrate the pro-oncogenic role of GPR55, we generated tumor xenografts by subcutaneous injection of shControl-PDV cells and shGPR55-PDV cells. We observed no differences in tumor incidence (100% in all cases) or latency, but receptor knockdown dramatically decreased tumor growth (Figure 5e). Collectively, these results demonstrate that GPR55 enhances the aggressiveness of skin cancer cells.
Finally, we analyzed GPR55 expression in human samples. GPR55 mRNA levels were hardly detectable in the normal skin (Figures 6a and b), but, interestingly, they were significantly increased in skin carcinomas compared with healthy tissue (Figures 6a and b). Similar results were found in other human squamous cell carcinomas such as larynx and oral cavity (Figures 6c–e) carcinomas, in which cancer tissue expressed significantly higher GPR55 mRNA levels than the corresponding non-tumor tissue. Oral carcinoma data were obtained from a previously published microarray dataset,13 and in this case Cyclin D1 and c-Fos tumor expression were also available. Of interest, tumor GPR55 expression positively correlated with tumor Cyclin D1 (Figure 6f) and c-Fos expression (Figure 6g), further supporting the link between GPR55 and cancer cell proliferation.
The orphan receptor GPR55 was identified and cloned in 1999.14 Experiments performed with GPR55-deficient mice have demonstrated that it participates in the control of inflammatory and neuropathic pain,11 and bone metabolism,10 but the broad distribution of this receptor throughout the body suggests that it may modulate many more physio-pathological functions.15 Regarding cancer, recent data suggest that GPR55 may be part of the molecular circuitry controlling tumor growth. Thus, this receptor promotes glioma, breast,7 prostate and ovarian8 cancer cell proliferation, and breast cancer cell migration6 in culture. It also induces tumor growth in a xenograft-based model of glioma.7 Here, by using a well-established model of chemically-induced carcinogenesis, we demonstrate that GPR55 has a prominent role in malignant growth. Specifically, we show that GPR55 deficiency makes mice significantly resistant to two-stage skin carcinogenesis. The main changes observed between WT and GPR55 KO mice concerned tumor multiplicity and not tumor incidence or tumor latency. We have demonstrated a link between GPR55 expression and increased cell proliferation. Thus, TPA- induced epidermal hyperproliferation in vivo is accompanied by an increase in GPR55 levels and, most important, is not evident in GPR55-deficient mice. Additionally, the cells undergoing proliferation in response to TPA are those with increased GPR55 expression. Moreover, when GPR55 is knocked down in skin cancer cells, their growth ability is hampered. Both orphan and well-characterized GPCRs have been previously associated with different aspects of cancer development, including aberrant cancer cell proliferation.16,17 Prominent examples of such GPCRs are lysophosphatidic acid (LPA) receptors. LPA is a lysophos- pholipid with potent mitogenic activity that produces its biological effects by binding to six specific GPCRs, namely LPA1-6.18,19 LPA signaling is frequently overactivated in human tumors and it is currently accepted to contribute to cancer progression by stimulating, among others, cancer cell proliferation.16,18 The similarities between LPA receptors and GPR55 are abundant. First, they are two of the few GPCRs engaged by lipid ligands. Although the pharmacology of GPR55 is still quite controversial, several reports have consistently demonstrated that GPR55 can be activated by LPI.1,10,20–25 Interestingly, LPI, as LPA, has mitogenic properties. Specifically, it has been described that epithelial cells4 and fibroblasts5 generate proliferation-inducing LPI upon ras-driven transformation. Cannabinoids, the active components of marijuana and their derivatives, which also have lipid nature, seem to activate this receptor as well, but in this case the evidence is not as consistent as for LPI: some cannabinoids were active in some reports and inactive in others, some were agonists in some studies and antagonists in others, and so on.26 Another interesting similarity between LPA receptors and GPR55 is that their respective ligands are upregulated in cancer. Thus, LPA is elevated in ascites27 and plasma28 of ovarian cancer patients and in the plasma of hepatocellular carcinoma patients.29 Regarding GPR55 ligands, LPI levels are increased in ascites3 and plasma2 from patients with ovarian cancer, and the endo- genous ligands of cannabinoid receptors (endocannabinoids) are enhanced in tumor vs non-tumor tissue in brain,30,31 prostate, endometrial32 and colorectal33 cancer patients. Together with their ligands, both LPA and GPR55 receptors themselves are frequently upregulated in neoplasias. LPA receptors, LPA2 in particular, are aberrantly expressed in a wide variety of human tumors, including thyroid,34 ovarian,35 colorectal,36 breast,37 colon, lung, uterus, rectum, testis,38 gastric39 and prostate40 carcinomas. The same is true for GPR55: herein, we present data showing that GPR55 is elevated in human carcinomas of the skin, larynx and oral cavity compared with the corresponding non-tumor tissues. These observations are in line with previous reports demonstrating that GPR55 mRNA is present in a wide collection of human cancer cell lines from breast,6,7 brain, cervix, skin, pancreas, liver,7 ovary, prostate,8 bile ducts41 and hematological origin.7,23,42 Moreover, the levels of this receptor are upregulated in breast, brain and pancreatic cancer compared with the corresponding healthy tissue, and correlate with tumor aggressiveness, that is, higher histological grades and, in the case of gliomas, lower survival rates.7
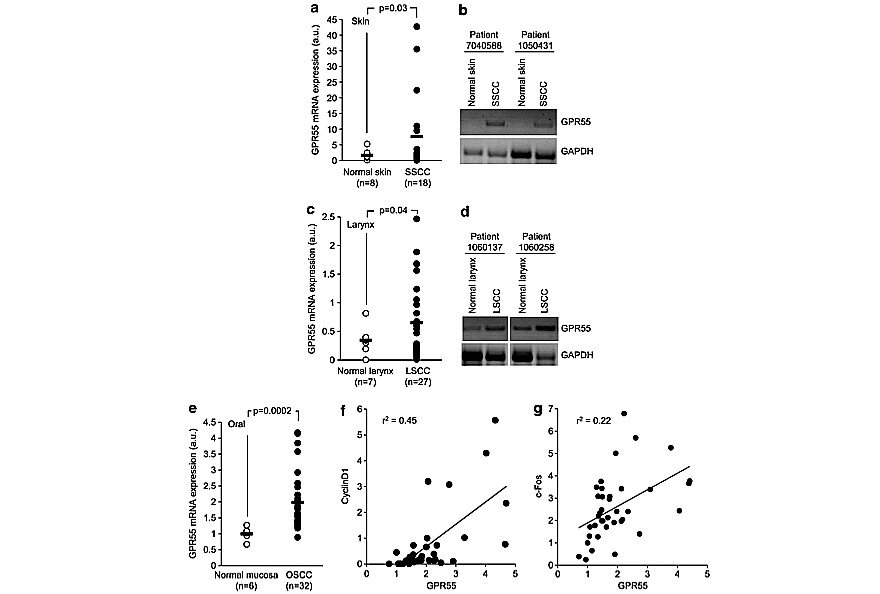
E Pe ́rez-Go ́mez et al
Figure 6. Expression of GPR55 in human samples. GPR55 mRNA levels were determined in a collection of skin- (a and b) and larynx-related (c and d) human samples. (a and c), GPR55 mRNA levels as determined by RTQ–PCR. (b and d) Representative semi-quantitative PCRs corresponding to pairs of tumor and non-tumor samples from the same patients. GPR55, c-Fos and Cyclin D1 mRNA expression data in oral cavity (e–g) were obtained from the microarray data set published in GEO database with the accession number GSE10121. (e) GPR55 mRNA levels in non-tumor and tumor samples. f and g represent GPR55 expression vs cyclin D1 and c-Fos expression, respectively. Results are expressed in arbitrary units. r2 1⁄4 coefficient of determination (Pearson correlation coefficient2). SSCC, skin squamous cell carcinoma; LSCC, larynx squamous cell carcinoma; OSCC, oral squamous cell carcinoma.
Oncogene (2013) 2534 – 2542
MATERIALS AND METHODS
Animals and treatments
All procedures involving animals were performed with the approval of the Complutense University Animal Experimentation Committee in compliance with the European official regulations. GPR55 KO mice (B6;129S- Gpr55tm1Lex/Mmnc) were generated by Lexicon Pharmaceuticals (The Woodlands, TX, USA) and were obtained through the Mutant Mouse
Regional Resource Centers. This strain has a 129/SvEvBrd x C57BL6/J-mixed genetic background. As pure C57BL6/J mice are refractory to DMBA/ TPA-induced carcinogenesis,9 GPR55 KO animals were bred to the sensitive 129/Ola mice. Heterozygous animals from these progeny were intercrossed to generate the WT and GPR55 KO littermates used in this study. For the two-stage carcinogenesis experiments, tumors were induced on the shaved dorsal skin of WT (n 1⁄4 11) and GPR55 KO (n 1⁄4 11) mice by topical application of a single dose of DMBA (32 mg in 200 ml of acetone), followed by twice-weekly applications of TPA (12.5mg in 200ml of acetone) for 22 weeks. The number of tumors (42mm in diameter) was recorded weekly. TPA treatment was ended after 22 weeks, and animals were left untreated until week 38 after DMBA initiation to allow the development of carcinomas. Tumors were collected at different time points and histologically typed as previously described.12 For the acute TPA experiments, a single dose of TPA (12.5 mg) or the corresponding vehicle (200 ml of acetone) was applied onto the shaved dorsal skin of WT (n 1⁄4 10) and GPR55 KO (n 1⁄4 10) littermates. At the end of the experiments, mice were killed and tumors or skin were collected. Samples were divided into portions that were (1) frozen in Tissue-Tek (Sakura Finetek Europe, Zoeterwoude, The Netherlands) for immunofluorescence staining, (2) fixed in formalin for immunofluorescence, immunohistochemistry and H&E staining, or (3) snap-frozen for protein and RNA extraction. Samples were stored at -80 degrees C until analysis (except formalin-fixed samples, which were kept at room temperature.
Cell cultures, plasmids and transfections
The origin and culture conditions of the PDV-transformed murine keratinocytes has been described elsewhere.12 To stably knockdown GPR55, double-stranded oligonucleotides encoding shRNA against mouse GPR55 were inserted into the psi-U6-EGFP vector (GeneCopoeia, Rockville, MD, USA). A psi-U6-EGFP-unrelated sequence vector was used as control. Transfections were carried out using Lipofectamine-plus (Invitrogen, Barcelona, Spain) according to the manufacturer’s instructions. Cells were selected with puromycin (Sigma, St Louis, MO, USA), and individual clones were isolated using a FACS Vantage sorter (Becton Dickinson, Madrid, Spain). Cell viability was determined in 10% FBS (fetal bovine serum)- containing cell cultures by the MTT test (Sigma) according to manufacturer’s instructions.
Anchorage-independent growth
Cell invasion
Cells (2.5 x 104) were suspended in 500 ml DMEM supplemented with 0.1% FBS and loaded onto the upper compartment of BD BioCoat Matrigel Invasion Chambers (BD Biosciences, Bedford, MA, USA). FBS (5%) was used as chemoattractant in the lower compartment. Cell invasion was quantified by staining migrated cell nuclei with DAPI (40,6-diamidino-2-phenylindole) (Roche Applied Science, Barcelona, Spain).
Subcutaneous xenografts
For tumorigenicity assays, 106 viable cells were subcutaneously injected into the flank of 6-week-old athymic mice (Harlan Interfauna Iberica, Barcelona, Spain). Tumors were routinely measured and volume was calculated as (4p/3) x (width/2)2 x (length/2). Fifty days after tumor detection, animals were killed and tumors were collected and processed as described above.
Immunofluoresce analysis
Tissue-Tek or paraffin-embedded sections were fixed in 10% paraformal- dehyde and were subjected to heat-induced antigen retrieval in citrate buffer before exposure to primary antibodies. Anti-GPR55 antibody, the corresponding blocking peptide and anti-proliferating cell nuclear antigen antibody were from Abcam (Cambridge, UK). Anti-CD45R(B220) Alexa Fluor 647 antibody was from BD Biosciences. Secondary anti-rabbit antibodies Alexa Fluor 594 and Alexa Fluor 488 were from Invitrogen. Cell nuclei were stained with DAPI (Invitrogen). Fluorescence confocal images were acquired by using Leica TCS-SP2 software (Leica, Wetzlar, Germany).
Paraffin-embedded sections from tumors were deparaffinized and rehydrated using standard protocols and subjected to heat-induced antigen retrieval in citrate buffer. Endogenous peroxidase blockade and unspecific binding reduction were achieved by successive incubation with 3% H2O2 and 5% goat serum (Abcam) supplemented with 3% BSA and 1% Triton X-100 (Sigma), respectively. Samples were then incubated with anti- GPR55 antibody and further processed with appropriate secondary antibodies following the LSAB2 horseradish peroxidase kit’s instructions (Dako cytomation, Carpenteria, CA, USA). Images were acquired with Cell^A software (Olympus, Mu ̈nster, Germany)
Human tumor samples
Two series of skin (18 squamous cell carcinomas and 8 non-tumor skin specimens) and larynx samples (27 carcinomas and 7 non-tumor tissues) were obtained from the CNIO Tumor Bank Core Unit (Madrid, Spain). An additional data set corresponding to 32 oral squamous cell carcinomas plus 6 normal mucosas13 was analyzed. In this case, microarray data were obtained from GEO database, with the accession number GSE10121.
RT– and real-time quantitative–PCR
RNA was isolated with Trizol Reagent (Invitrogen) with the Real Star Kit (Durviz, Valencia, Spain), and cDNA was obtained with Transcriptor Reverse Transcriptase (Roche Applied Science). The primers used for RT– and RTQ (real-time quantitative)–PCR are in Supplementary Table 1. For RTQ–PCR, probes were from the Universal Probe Library (Roche Applied Science) and multispecies 18S RNA was used as reference. For RT–PCR, GAPDH was used as reference.
Western blot analysis
Cell lysates from tumors and cell lines were subjected to SDS–PAGE, and proteins transferred onto PVDF membranes. Blots were incubated with the following antibodies: anti-GPR55 (Abcam), anti-phospho-ERK (Thr202/ Tyr204), anti-ERK, anti-phospho-Akt (Ser473), cyclin D1 (Cell Signaling Technology, Danvers, MA, USA) and anti-a-tubulin (Sigma). Luminograms were obtained with the Amersham Enhanced Chemiluminescence Detection Kit (GE Healthcare, Uppsala, Sweden) and densitometric analysis was performed with Quantity One software (Bio-Rad, Madrid, Spain).
Statistical analysis
ANOVA with a post-hoc analysis by the Student–Newman–Keuls’ test
was routinely used. Unless otherwise stated, data are expressed as mean±s.e.m.
The authors declare no conflict of interest.
ACKNOWLEDGEMENTS
We are indebted to the members of our laboratories and to Dr MM Caffarel for critical discussion on this work. We thank Dr Manuel M Morente, Head of the CNIO Tumor Bank Core Unit, for kindly provided us with human tumor samples. This work was supported by grants from Fondo de Investigaciones Sanitarias (PI080253 to CS), Fundacio ́ n Mutua Madrilen ̃ a (to CS), GW Pharmaceuticals/Otsuka Pharmaceuticals (to CS), Comunidad de Madrid (S2010/BMD-2038 to MG) and Spanish Ministry of Science and Innovation (SAF2010-19152 to MQ and SAF2008-00121 and SAF2011-26122-C02- 01 to JMP). EPG and CA were the recipients of a Postdoctoral Research Contract from Fundacio ́n Cient ́ıfica Asociacio ́n Espan ̃ola Contra el Ca ́ncer (AECC) and a PFIS PhD studentship from the Spanish Ministry of Science and Innovation, respectively.
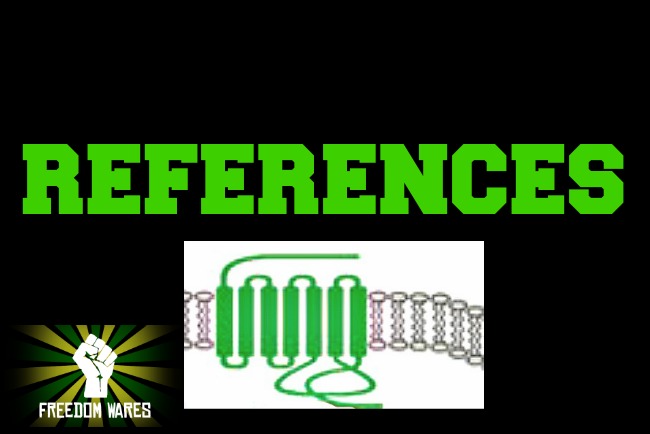